User login
Prevalence of Staphylococcus aureus and Use of Antistaphylococcal Therapy in Children Hospitalized with Pneumonia
Although Staphylococcus aureus pneumonia is common in children with cystic fibrosis and those with healthcare-associated infections (eg, ventilator-associated pneumonia),1,2 S. aureus is an uncommon cause of community-acquired pneumonia in children. In recent years, concerns have arisen about the increasing frequency and severity of staphylococcal pneumonia, largely fueled by the emergence of community-associated methicillin-resistant S. aureus (MRSA).3,4 Thus, therapy with clindamycin or vancomycin, both active against MRSA, has been recommended when S. aureus is suspected.5 Given the lack of rapid and sensitive approaches to the detection of the etiologies of pneumonia, antibiotic selection is most often empirical, contributing to overuse of anti-MRSA antibiotics. In addition, resistance against these antibiotics, especially clindamycin, has been increasing.6,7
A better understanding of the likelihood of staphylococcal pneumonia would help to optimize empirical antibiotic selection, allowing for judicious use of antistaphylococcal antibiotics, while also avoiding poor outcomes due to delays in effective treatment when S. aureus is present.8 Using data from a multicenter, population-based study of pneumonia hospitalizations in children, we sought to describe the prevalence, clinical characteristics, and in-hospital outcomes of staphylococcal pneumonia and the prevalence of antistaphylococcal antibiotic use.
METHODS
The Etiology of Pneumonia in the Community (EPIC) study was a prospective, active, population-based surveillance study of pneumonia hospitalizations among children (age <18 years) conducted between 2010 and 2012 at three children’s hospitals, including two in Tennessee and one in Utah.9 Children hospitalized with clinical evidence of pneumonia and radiographic evidence confirmed by a blinded review by study radiologists were enrolled. Etiologic assessments included blood analysis for bacterial culture, serology for eight respiratory viruses, pneumococcal and group A streptococcal polymerase chain reaction (PCR), and naso/oro-pharyngeal swabs for PCR for 13 respiratory viruses, Mycoplasma pneumoniae, and Chlamydophila pneumoniae. Data from other clinical specimens (pleural fluid, high-quality endotracheal aspirate, or quantified bronchoalveolar lavage fluid) were also recorded. For this study, we included only children with at least one bacterial culture and complete information about antibiotic use. Those with confirmed fungal pneumonia were excluded. Additional details regarding the study population and methods have been published previously.9
Staphylococcal pneumonia was defined based on the detection of S. aureus by culture (any site) or PCR (pleural fluid only), regardless of codetection of other pathogens. Antibiotic susceptibility profiles were used to classify S. aureus isolates as MRSA or methicillin-sensitive S. aureus (MSSA). The remaining children were classified as nonstaphylococcal pneumonia including children with other bacterial pathogens detected (classified as other bacterial pneumonia, excludes atypical bacteria), atypical bacteria, viruses, and no pathogens detected.
Use of anti-MRSA antibiotics (vancomycin, clindamycin, linezolid, doxycycline, and trimethoprim-sulfamethoxazole) and any antistaphylococcal antibiotics (anti-MRSA agents plus oxacillin, nafcillin, and cefazolin) during and after the first two calendar days of admission was identified by medical record review.
Descriptive statistics included number (%) and median (interquartile range, [IQR]) for categorical and continuous variables, respectively. Baseline clinical characteristics and outcomes were compared between children with staphylococcal versus nonstaphylococcal pneumonia, those with staphylococcal versus other bacterial pneumonia, and those with MRSA versus MSSA pneumonia using Wilcoxon rank-sum and Pearson’s chi-square tests where appropriate. To account for multiple comparisons, we used a Bonferroni corrected P value threshold of <.001 to determine statistical significance.
RESULTS
Of the 2,358 children enrolled in the EPIC study hospitalized with radiographically confirmed pneumonia, 2,146 (91.0%) had ≥1 bacterial culture obtained. Two children with Histoplasma capsulatum fungal infection and six children with incomplete antibiotic utilization data were excluded, yielding a final study population of 2,138 children. Among these, blood samples were obtained from 2,134 (>99%) children for culture, pleural fluid from 87 (4%) children, bronchoalveolar lavage fluid from 31 (1%) children, and endotracheal aspirate from 80 (4%) children. Across all culture types, there were 2,332 initial cultures; 2,150 (92%) were collected within the first 24 hours.
Staphylococcal pneumonia was detected in 23 of the 2,138 children (1% [95% CI 0.7, 1.6]; 17 MRSA, 6 MSSA). Of these, 6/23 (26%) had bacteremia, 12/23 (52%) had a positive pleural fluid, and 9/23 (39%) had a positive culture from bronchoalveolar lavage fluid or endotracheal aspirate; 4/23 (17%) children had S. aureus detected from more than one site. Three children (13%) with S. aureus had a viral codetection, including two with influenza.
Compared with children with nonstaphylococcal pneumonia, those with staphylococcal pneumonia were more likely to have a parapneumonic effusion (78% vs 12%, P < .001), but less likely to have cough (78% vs 95%, P < .001). Other baseline characteristics were similar between the two groups. Children with staphylococcal pneumonia had more adverse outcomes than those without (Table), including longer median length of stay (10 vs 3 days, P < .001), more frequent admission to intensive care (83% vs 21%, P < .001), and more frequent invasive mechanical ventilation (65% vs 7%, P < .001). Similar findings were noted when staphylococcal pneumonia was compared with pneumonia caused due to other bacterial pathogens (n = 124). There were no significant differences in baseline characteristics or clinical course between children with MRSA and MSSA pneumonia, although the numbers were small. Overall, S. aureus was detected in 18/267 (7%) children with parapneumonic effusion and 19/462 (4%) children admitted to intensive care. Importantly, there were no confirmed S. aureus cases among children with less severe pneumonia, defined as lacking both parapneumonic effusion and intensive care admission (n = 1,488).
Overall, 519 children (24%) received antistaphylococcal therapy during their hospitalization (512/519, 99% received anti-MRSA therapy), including 22 of the 23 children with S. aureus detected (the only child without antistaphylococcal therapy had S. aureus detected from a high-quality endotracheal tube aspirate only and also had respiratory syncytial virus detected). Clindamycin was most often used (n = 266, 51%), followed by vancomycin (n = 128, 24%), clindamycin plus vancomycin (n = 83, 16%), and others (n = 42, 8%). During the first two days of hospitalization, 479 children (22%) received antistaphylococcal therapy (477 received anti-MRSA therapy). After the first two days, 351 children (16%) received antistaphylococcal therapy (346/351, 99% received anti-MRSA therapy). Use of antistaphylococcal therapy was very common in those admitted to intensive care (182/462, 39%; all but two received anti-MRSA therapy) and in those requiring invasive mechanical ventilation (103/159, 65%). Among those lacking both parapneumonic effusion and intensive care admission (n = 1488), 232 (16%) received antistaphylococcal therapy.
DISCUSSION
In our large, population-based study of >2,000 children hospitalized with community-acquired pneumonia, S. aureus was identified in only 1% of children. Compared with children with other pneumonia etiologies, staphylococcal pneumonia was associated with increased disease severity. Among the small numbers studied, no differences in outcomes were found between children with MRSA and MSSA disease. Despite the low prevalence of staphylococcal pneumonia, almost 1 in 4 children received antistaphylococcal antibiotic therapy; anti-MRSA therapy was used almost exclusively.
The severity of staphylococcal pneumonia was striking, with >80% of children with S. aureus detected being admitted to intensive care, about 65% requiring invasive mechanical ventilation, and >75% with parapneumonic effusion. These findings are similar to those of prior retrospective studies.4,10 The association between staphylococcal pneumonia and adverse outcomes underscores the importance of prompt institution of antimicrobial therapy targeting S. aureus in high-risk patients. This is noteworthy given recent epidemiological data demonstrating increases in MSSA relative to MRSA infections in children,6 and the known superiority of beta-lactam versus vancomycin for MSSA infections, including pneumonia.11
Although detection of staphylococcal infection was rare, almost a quarter of children received antistaphylococcal therapy; nearly all of these children received anti-MRSA therapy. Confirming a bacterial etiology of pneumonia, however, is challenging. Given the severity associated with staphylococcal pneumonia, it is not surprising that use of antistaphylococcal therapy outpaced staphylococcal detections. Antistaphylococcal therapy was especially common in those with severe pneumonia, suggesting that disease severity is an important factor that influences initial antibiotic treatment decisions. Even so, two children with MRSA detected did not initially receive anti-MRSA therapy, highlighting the challenge of balancing judicious antibiotic selection along with ensuring effective treatment. Perhaps more striking is the finding that 16% of children received antistaphylococcal therapy beyond the first two days of hospitalization, presumably after the initial culture results were available. This suggests that clinicians are reluctant to stop antistaphylococcal therapy when the etiology is unknown, although certain features, such as negative cultures, rapid clinical improvement, and lack of risk factors for staphylococcal disease, may provide important clues to support de-escalation of empiric antibiotic therapy. It is also possible that some antibiotics with antistaphylococcal activity were used for alternative indications (eg, clindamycin for penicillin allergy or concern for aspiration pneumonia).
A simple strategy for tailoring antibiotic treatment is maximizing opportunities to identify a causative pathogen. Despite the very low yield of blood cultures in children with pneumonia overall, bacteremia is more common in children with severe pneumonia and those with parapneumonic effusion, especially when cultures are obtained prior to antibiotic use.12,13 Similarly, obtaining pleural fluid is often therapeutic and significantly improves the chances of identifying a bacterial pathogen.14 Moreover, at least one study suggests that S. aureus is much less likely in cases of culture-negative parapneumonic effusions.15 Institutional guidelines, order sets, and antimicrobial stewardship teams are also effective strategies that can facilitate judicious antibiotic use. In particular, stewardship experts can be very useful in assisting clinicians around de-escalation of therapy.16 Use of procalcitonin, a biomarker associated with bacterial infections,17 and prognostic tools to identify risk for adverse outcomes,18 may also inform treatment decisions and are deserving of further study.
Our study must be considered in
Our study demonstrates a very low prevalence of S. aureus detection among children hospitalized with pneumonia and highlights the association between staphylococcal disease and adverse in-hospital outcomes. We also document important discrepancies between disease prevalence and utilization of antistaphylococcal therapy, especially anti-MRSA therapy. Improved approaches are needed to minimize overuse of antistaphylococcal antibiotics while also ensuring adequate therapy for those who need it.
Disclosures
Drs. Zhu, Edwards, Self, Ampofo, Arnold, McCullers, and Williams report grants from the Centers for Disease Control and Prevention during the conduct of the study. Ms. Frush has nothing to disclose. Dr. Jain has nothing to disclose. Dr. Grijalva reports other from Merck, grants and other from Sanofi, other from Pfizer, grants from CDC, grants from AHRQ, grants from NIH, and grants from Campbell Alliance, outside the submitted work. Dr. Self reports grants from CDC, during the conduct of the study; personal fees from Cempra Pharmaceuticals, grants and personal fees from Ferring Pharmaceuticals, personal fees from BioTest AG, personal fees from Abbott Point of Care, personal fees from Gilead Pharmaceuticals, personal fees from Pfizer, grants from Merck, outside the submitted work. Dr. Thomsen has nothing to disclose. Dr. Ampofo reports grants from CDC, during the conduct of the study; other from GlaxoSmithKline, other from Cubist Pharmaceuticals outside the submitted work; and KA collaborate with BioFire Diagnostics, Inc. (formerly Idaho Technology, Inc.) on several NIH grants. Dr. Pavia reports grants from NAID/NIH, grants from NAID/NIH, grants from CDC, personal fees from WebMD, personal fees from Antimicrobial Therapy Inc., outside the submitted work.
Funding
This work was supported by the National Institute of Allergy and Infectious Diseases of the National Institutes of Health under Award Number K23AI104779 to D.J.W. and Award 1K23AI113150 to I.P.T., the National Institute of General Medical Sciences under Award K23GM110469 to W.H.S., and the Agency for Healthcare Research and Quality under Award R03HS022342 to C.G.G. The EPIC study was supported by the Influenza Division in the National Center for Immunizations and Respiratory Diseases at the Centers for Disease Control and Prevention through cooperative agreements with each study site and was based on a competitive research funding opportunity. The findings and conclusions in this report are those of the authors and do not necessarily represent the views of the National Institute of Allergy and Infectious Diseases, the National Institute of General Medical Sciences, the Agency for Healthcare Research and Quality, or the Centers for Disease Control and Prevention.
1. Akil N, Muhlebach MS. Biology and management of methicillin resistant Staphylococcus aureus in cystic fibrosis. Pediatr Pulmonol. 2018. doi: 10.1002/ppul.24139. PubMed
2. Srinivasan R, Asselin J, Gildengorin G, Wiener-Kronish J, Flori HR. A prospective study of ventilator-associated pneumonia in children. Pediatrics.
2009;123(4):1108-1115. doi: 10.1542/peds.2008-1211. PubMed
3. Gonzalez BE, Martinez-Aguilar G, Hulten KG, et al. Severe Staphylococcal sepsis in adolescents in the era of community-acquired methicillin-resistant Staphylococcus aureus. Pediatrics. 2005;115(3):642-648. doi: 10.1542/peds.2004-2300. PubMed
4. Carrillo-Marquez MA, Hulten KG, Hammerman W, Lamberth L, Mason EO, Kaplan SL. Staphylococcus aureus pneumonia in children in the era of community-acquired methicillin-resistance at Texas Children’s Hospital. Pediatr Infect Dis J. 2011;30(7):545-550. doi: 10.1097/INF.0b013e31821618be. PubMed
5. Bradley JS, Byington CL, Shah SS, et al. The management of community-acquired pneumonia in infants and children older than 3 months of age: clinical practice guidelines by the pediatric infectious diseases society and the infectious diseases society of America. Clin Infect Dis. 2011;53(7):e25-e76. doi: 10.1093/cid/cir531. PubMed
6. Sutter DE, Milburn E, Chukwuma U, Dzialowy N, Maranich AM, Hospenthal DR. Changing susceptibility of Staphylococcus aureus in a US pediatric population. Pediatrics. 2016;137(4):e20153099–e20153099. doi: 10.1542/peds.2015-3099. PubMed
7. Sakoulas G, Moellering RC, Jr. Increasing antibiotic resistance among methicillin-resistant Staphylococcus aureus strains. Clin Infect Dis. 2008;46(Suppl 5):S360-S367. doi: 10.1086/533592. PubMed
8. Rubinstein E, Kollef MH, Nathwani D. Pneumonia caused by methicillin-resistant
Staphylococcus aureus. Clin Infect Dis. 2008;46(Suppl 5):S378-S385. doi: 10.1086/533594. PubMed
9. Jain S, Williams DJ, Arnold SR, et al. Community-acquired pneumonia requiring hospitalization among U.S. children. N Engl J Med. 2015;372(9):835-845. doi: 10.1056/NEJMoa1405870. PubMed
10. Kallen AJ, Reed C, Patton M, Arnold KE, Finelli L, Hageman J. Staphylococcus aureus community-onset pneumonia in patients admitted to children’s hospitals during autumn and winter of 2006-2007. Epidemiol Infect. 2010;138(5):666-672. doi: 10.1017/S095026880999135X. PubMed
11. González C, Rubio M, Romero-Vivas J, González M, Picazo JJ. Bacteremic pneumonia due to Staphylococcus aureus: A comparison of disease caused by methicillin-resistant and methicillin-susceptible organisms. Clin Infect Dis. 1999;29(5):1171-1177. doi: 10.1086/313440. PubMed
12. Myers AL, Hall M, Williams DJ, et al. Prevalence of bacteremia in hospitalized pediatric patients with community-acquired pneumonia. Pediatr Infect Dis J. 2013;32(7):736-740. doi: 10.1097/INF.0b013e318290bf63. PubMed
13. Iroh Tam PY, Bernstein E, Ma X, Ferrieri P. Blood culture in evaluation of pediatric community-acquired pneumonia: A systematic review and meta-analysis. Hosp Pediatr. 2015;5(6):324-336. doi: 10.1542/hpeds.2014-0138. PubMed
14. Byington CL, Spencer LY, Johnson TA, et al. An epidemiological investigation of a sustained high rate of pediatric parapneumonic empyema: risk factors and microbiological associations. Clin Infect Dis. 2002;34(4):434-440. doi: 10.1086/338460. PubMed
15. Blaschke AJ, Heyrend C, Byington CL, et al. Molecular analysis improves pathogen identifi cation and epidemiologic study of pediatric parapneumonic empyema. Pediatr Infect Dis J. 2011;30(4):289-294. doi: 10.1097/INF.0b013e3182002d14. PubMed
16. Banerjee R, Teng CB, Cunningham SA, et al. Randomized trial of rapid multiplex polymerase chain reaction-based blood culture identifi cation and susceptibility testing. Clin Infect Dis. 2015;61(7):1071-1080. doi: 10.1093/cid/civ447. PubMed
17. Stockmann C, Ampofo K, Killpack J, et al. Procalcitonin accurately identifies hospitalized children with low risk of bacterial community-acquired pneumonia. J Pediatr Infect Dis Soc. 2018;7(1):46–53. doi: 10.1093/jpids/piw091. PubMed
18. Williams DJ, Zhu Y, Grijalva CG, et al. Predicting severe pneumonia outcomes in children. Pediatrics. 2016;138(4). doi: 10.1542/peds.2016-1019. PubMed
19. Brogan TV, Hall M, Williams DJ, et al. Variability in processes of care and outcomes among children hospitalized with community-acquired pneumonia. Pediatr Infect Dis J. 2012;31(10):1036-1041. doi: 10.1097/INF.0b013e-31825f2b10. PubMed
Although Staphylococcus aureus pneumonia is common in children with cystic fibrosis and those with healthcare-associated infections (eg, ventilator-associated pneumonia),1,2 S. aureus is an uncommon cause of community-acquired pneumonia in children. In recent years, concerns have arisen about the increasing frequency and severity of staphylococcal pneumonia, largely fueled by the emergence of community-associated methicillin-resistant S. aureus (MRSA).3,4 Thus, therapy with clindamycin or vancomycin, both active against MRSA, has been recommended when S. aureus is suspected.5 Given the lack of rapid and sensitive approaches to the detection of the etiologies of pneumonia, antibiotic selection is most often empirical, contributing to overuse of anti-MRSA antibiotics. In addition, resistance against these antibiotics, especially clindamycin, has been increasing.6,7
A better understanding of the likelihood of staphylococcal pneumonia would help to optimize empirical antibiotic selection, allowing for judicious use of antistaphylococcal antibiotics, while also avoiding poor outcomes due to delays in effective treatment when S. aureus is present.8 Using data from a multicenter, population-based study of pneumonia hospitalizations in children, we sought to describe the prevalence, clinical characteristics, and in-hospital outcomes of staphylococcal pneumonia and the prevalence of antistaphylococcal antibiotic use.
METHODS
The Etiology of Pneumonia in the Community (EPIC) study was a prospective, active, population-based surveillance study of pneumonia hospitalizations among children (age <18 years) conducted between 2010 and 2012 at three children’s hospitals, including two in Tennessee and one in Utah.9 Children hospitalized with clinical evidence of pneumonia and radiographic evidence confirmed by a blinded review by study radiologists were enrolled. Etiologic assessments included blood analysis for bacterial culture, serology for eight respiratory viruses, pneumococcal and group A streptococcal polymerase chain reaction (PCR), and naso/oro-pharyngeal swabs for PCR for 13 respiratory viruses, Mycoplasma pneumoniae, and Chlamydophila pneumoniae. Data from other clinical specimens (pleural fluid, high-quality endotracheal aspirate, or quantified bronchoalveolar lavage fluid) were also recorded. For this study, we included only children with at least one bacterial culture and complete information about antibiotic use. Those with confirmed fungal pneumonia were excluded. Additional details regarding the study population and methods have been published previously.9
Staphylococcal pneumonia was defined based on the detection of S. aureus by culture (any site) or PCR (pleural fluid only), regardless of codetection of other pathogens. Antibiotic susceptibility profiles were used to classify S. aureus isolates as MRSA or methicillin-sensitive S. aureus (MSSA). The remaining children were classified as nonstaphylococcal pneumonia including children with other bacterial pathogens detected (classified as other bacterial pneumonia, excludes atypical bacteria), atypical bacteria, viruses, and no pathogens detected.
Use of anti-MRSA antibiotics (vancomycin, clindamycin, linezolid, doxycycline, and trimethoprim-sulfamethoxazole) and any antistaphylococcal antibiotics (anti-MRSA agents plus oxacillin, nafcillin, and cefazolin) during and after the first two calendar days of admission was identified by medical record review.
Descriptive statistics included number (%) and median (interquartile range, [IQR]) for categorical and continuous variables, respectively. Baseline clinical characteristics and outcomes were compared between children with staphylococcal versus nonstaphylococcal pneumonia, those with staphylococcal versus other bacterial pneumonia, and those with MRSA versus MSSA pneumonia using Wilcoxon rank-sum and Pearson’s chi-square tests where appropriate. To account for multiple comparisons, we used a Bonferroni corrected P value threshold of <.001 to determine statistical significance.
RESULTS
Of the 2,358 children enrolled in the EPIC study hospitalized with radiographically confirmed pneumonia, 2,146 (91.0%) had ≥1 bacterial culture obtained. Two children with Histoplasma capsulatum fungal infection and six children with incomplete antibiotic utilization data were excluded, yielding a final study population of 2,138 children. Among these, blood samples were obtained from 2,134 (>99%) children for culture, pleural fluid from 87 (4%) children, bronchoalveolar lavage fluid from 31 (1%) children, and endotracheal aspirate from 80 (4%) children. Across all culture types, there were 2,332 initial cultures; 2,150 (92%) were collected within the first 24 hours.
Staphylococcal pneumonia was detected in 23 of the 2,138 children (1% [95% CI 0.7, 1.6]; 17 MRSA, 6 MSSA). Of these, 6/23 (26%) had bacteremia, 12/23 (52%) had a positive pleural fluid, and 9/23 (39%) had a positive culture from bronchoalveolar lavage fluid or endotracheal aspirate; 4/23 (17%) children had S. aureus detected from more than one site. Three children (13%) with S. aureus had a viral codetection, including two with influenza.
Compared with children with nonstaphylococcal pneumonia, those with staphylococcal pneumonia were more likely to have a parapneumonic effusion (78% vs 12%, P < .001), but less likely to have cough (78% vs 95%, P < .001). Other baseline characteristics were similar between the two groups. Children with staphylococcal pneumonia had more adverse outcomes than those without (Table), including longer median length of stay (10 vs 3 days, P < .001), more frequent admission to intensive care (83% vs 21%, P < .001), and more frequent invasive mechanical ventilation (65% vs 7%, P < .001). Similar findings were noted when staphylococcal pneumonia was compared with pneumonia caused due to other bacterial pathogens (n = 124). There were no significant differences in baseline characteristics or clinical course between children with MRSA and MSSA pneumonia, although the numbers were small. Overall, S. aureus was detected in 18/267 (7%) children with parapneumonic effusion and 19/462 (4%) children admitted to intensive care. Importantly, there were no confirmed S. aureus cases among children with less severe pneumonia, defined as lacking both parapneumonic effusion and intensive care admission (n = 1,488).
Overall, 519 children (24%) received antistaphylococcal therapy during their hospitalization (512/519, 99% received anti-MRSA therapy), including 22 of the 23 children with S. aureus detected (the only child without antistaphylococcal therapy had S. aureus detected from a high-quality endotracheal tube aspirate only and also had respiratory syncytial virus detected). Clindamycin was most often used (n = 266, 51%), followed by vancomycin (n = 128, 24%), clindamycin plus vancomycin (n = 83, 16%), and others (n = 42, 8%). During the first two days of hospitalization, 479 children (22%) received antistaphylococcal therapy (477 received anti-MRSA therapy). After the first two days, 351 children (16%) received antistaphylococcal therapy (346/351, 99% received anti-MRSA therapy). Use of antistaphylococcal therapy was very common in those admitted to intensive care (182/462, 39%; all but two received anti-MRSA therapy) and in those requiring invasive mechanical ventilation (103/159, 65%). Among those lacking both parapneumonic effusion and intensive care admission (n = 1488), 232 (16%) received antistaphylococcal therapy.
DISCUSSION
In our large, population-based study of >2,000 children hospitalized with community-acquired pneumonia, S. aureus was identified in only 1% of children. Compared with children with other pneumonia etiologies, staphylococcal pneumonia was associated with increased disease severity. Among the small numbers studied, no differences in outcomes were found between children with MRSA and MSSA disease. Despite the low prevalence of staphylococcal pneumonia, almost 1 in 4 children received antistaphylococcal antibiotic therapy; anti-MRSA therapy was used almost exclusively.
The severity of staphylococcal pneumonia was striking, with >80% of children with S. aureus detected being admitted to intensive care, about 65% requiring invasive mechanical ventilation, and >75% with parapneumonic effusion. These findings are similar to those of prior retrospective studies.4,10 The association between staphylococcal pneumonia and adverse outcomes underscores the importance of prompt institution of antimicrobial therapy targeting S. aureus in high-risk patients. This is noteworthy given recent epidemiological data demonstrating increases in MSSA relative to MRSA infections in children,6 and the known superiority of beta-lactam versus vancomycin for MSSA infections, including pneumonia.11
Although detection of staphylococcal infection was rare, almost a quarter of children received antistaphylococcal therapy; nearly all of these children received anti-MRSA therapy. Confirming a bacterial etiology of pneumonia, however, is challenging. Given the severity associated with staphylococcal pneumonia, it is not surprising that use of antistaphylococcal therapy outpaced staphylococcal detections. Antistaphylococcal therapy was especially common in those with severe pneumonia, suggesting that disease severity is an important factor that influences initial antibiotic treatment decisions. Even so, two children with MRSA detected did not initially receive anti-MRSA therapy, highlighting the challenge of balancing judicious antibiotic selection along with ensuring effective treatment. Perhaps more striking is the finding that 16% of children received antistaphylococcal therapy beyond the first two days of hospitalization, presumably after the initial culture results were available. This suggests that clinicians are reluctant to stop antistaphylococcal therapy when the etiology is unknown, although certain features, such as negative cultures, rapid clinical improvement, and lack of risk factors for staphylococcal disease, may provide important clues to support de-escalation of empiric antibiotic therapy. It is also possible that some antibiotics with antistaphylococcal activity were used for alternative indications (eg, clindamycin for penicillin allergy or concern for aspiration pneumonia).
A simple strategy for tailoring antibiotic treatment is maximizing opportunities to identify a causative pathogen. Despite the very low yield of blood cultures in children with pneumonia overall, bacteremia is more common in children with severe pneumonia and those with parapneumonic effusion, especially when cultures are obtained prior to antibiotic use.12,13 Similarly, obtaining pleural fluid is often therapeutic and significantly improves the chances of identifying a bacterial pathogen.14 Moreover, at least one study suggests that S. aureus is much less likely in cases of culture-negative parapneumonic effusions.15 Institutional guidelines, order sets, and antimicrobial stewardship teams are also effective strategies that can facilitate judicious antibiotic use. In particular, stewardship experts can be very useful in assisting clinicians around de-escalation of therapy.16 Use of procalcitonin, a biomarker associated with bacterial infections,17 and prognostic tools to identify risk for adverse outcomes,18 may also inform treatment decisions and are deserving of further study.
Our study must be considered in
Our study demonstrates a very low prevalence of S. aureus detection among children hospitalized with pneumonia and highlights the association between staphylococcal disease and adverse in-hospital outcomes. We also document important discrepancies between disease prevalence and utilization of antistaphylococcal therapy, especially anti-MRSA therapy. Improved approaches are needed to minimize overuse of antistaphylococcal antibiotics while also ensuring adequate therapy for those who need it.
Disclosures
Drs. Zhu, Edwards, Self, Ampofo, Arnold, McCullers, and Williams report grants from the Centers for Disease Control and Prevention during the conduct of the study. Ms. Frush has nothing to disclose. Dr. Jain has nothing to disclose. Dr. Grijalva reports other from Merck, grants and other from Sanofi, other from Pfizer, grants from CDC, grants from AHRQ, grants from NIH, and grants from Campbell Alliance, outside the submitted work. Dr. Self reports grants from CDC, during the conduct of the study; personal fees from Cempra Pharmaceuticals, grants and personal fees from Ferring Pharmaceuticals, personal fees from BioTest AG, personal fees from Abbott Point of Care, personal fees from Gilead Pharmaceuticals, personal fees from Pfizer, grants from Merck, outside the submitted work. Dr. Thomsen has nothing to disclose. Dr. Ampofo reports grants from CDC, during the conduct of the study; other from GlaxoSmithKline, other from Cubist Pharmaceuticals outside the submitted work; and KA collaborate with BioFire Diagnostics, Inc. (formerly Idaho Technology, Inc.) on several NIH grants. Dr. Pavia reports grants from NAID/NIH, grants from NAID/NIH, grants from CDC, personal fees from WebMD, personal fees from Antimicrobial Therapy Inc., outside the submitted work.
Funding
This work was supported by the National Institute of Allergy and Infectious Diseases of the National Institutes of Health under Award Number K23AI104779 to D.J.W. and Award 1K23AI113150 to I.P.T., the National Institute of General Medical Sciences under Award K23GM110469 to W.H.S., and the Agency for Healthcare Research and Quality under Award R03HS022342 to C.G.G. The EPIC study was supported by the Influenza Division in the National Center for Immunizations and Respiratory Diseases at the Centers for Disease Control and Prevention through cooperative agreements with each study site and was based on a competitive research funding opportunity. The findings and conclusions in this report are those of the authors and do not necessarily represent the views of the National Institute of Allergy and Infectious Diseases, the National Institute of General Medical Sciences, the Agency for Healthcare Research and Quality, or the Centers for Disease Control and Prevention.
Although Staphylococcus aureus pneumonia is common in children with cystic fibrosis and those with healthcare-associated infections (eg, ventilator-associated pneumonia),1,2 S. aureus is an uncommon cause of community-acquired pneumonia in children. In recent years, concerns have arisen about the increasing frequency and severity of staphylococcal pneumonia, largely fueled by the emergence of community-associated methicillin-resistant S. aureus (MRSA).3,4 Thus, therapy with clindamycin or vancomycin, both active against MRSA, has been recommended when S. aureus is suspected.5 Given the lack of rapid and sensitive approaches to the detection of the etiologies of pneumonia, antibiotic selection is most often empirical, contributing to overuse of anti-MRSA antibiotics. In addition, resistance against these antibiotics, especially clindamycin, has been increasing.6,7
A better understanding of the likelihood of staphylococcal pneumonia would help to optimize empirical antibiotic selection, allowing for judicious use of antistaphylococcal antibiotics, while also avoiding poor outcomes due to delays in effective treatment when S. aureus is present.8 Using data from a multicenter, population-based study of pneumonia hospitalizations in children, we sought to describe the prevalence, clinical characteristics, and in-hospital outcomes of staphylococcal pneumonia and the prevalence of antistaphylococcal antibiotic use.
METHODS
The Etiology of Pneumonia in the Community (EPIC) study was a prospective, active, population-based surveillance study of pneumonia hospitalizations among children (age <18 years) conducted between 2010 and 2012 at three children’s hospitals, including two in Tennessee and one in Utah.9 Children hospitalized with clinical evidence of pneumonia and radiographic evidence confirmed by a blinded review by study radiologists were enrolled. Etiologic assessments included blood analysis for bacterial culture, serology for eight respiratory viruses, pneumococcal and group A streptococcal polymerase chain reaction (PCR), and naso/oro-pharyngeal swabs for PCR for 13 respiratory viruses, Mycoplasma pneumoniae, and Chlamydophila pneumoniae. Data from other clinical specimens (pleural fluid, high-quality endotracheal aspirate, or quantified bronchoalveolar lavage fluid) were also recorded. For this study, we included only children with at least one bacterial culture and complete information about antibiotic use. Those with confirmed fungal pneumonia were excluded. Additional details regarding the study population and methods have been published previously.9
Staphylococcal pneumonia was defined based on the detection of S. aureus by culture (any site) or PCR (pleural fluid only), regardless of codetection of other pathogens. Antibiotic susceptibility profiles were used to classify S. aureus isolates as MRSA or methicillin-sensitive S. aureus (MSSA). The remaining children were classified as nonstaphylococcal pneumonia including children with other bacterial pathogens detected (classified as other bacterial pneumonia, excludes atypical bacteria), atypical bacteria, viruses, and no pathogens detected.
Use of anti-MRSA antibiotics (vancomycin, clindamycin, linezolid, doxycycline, and trimethoprim-sulfamethoxazole) and any antistaphylococcal antibiotics (anti-MRSA agents plus oxacillin, nafcillin, and cefazolin) during and after the first two calendar days of admission was identified by medical record review.
Descriptive statistics included number (%) and median (interquartile range, [IQR]) for categorical and continuous variables, respectively. Baseline clinical characteristics and outcomes were compared between children with staphylococcal versus nonstaphylococcal pneumonia, those with staphylococcal versus other bacterial pneumonia, and those with MRSA versus MSSA pneumonia using Wilcoxon rank-sum and Pearson’s chi-square tests where appropriate. To account for multiple comparisons, we used a Bonferroni corrected P value threshold of <.001 to determine statistical significance.
RESULTS
Of the 2,358 children enrolled in the EPIC study hospitalized with radiographically confirmed pneumonia, 2,146 (91.0%) had ≥1 bacterial culture obtained. Two children with Histoplasma capsulatum fungal infection and six children with incomplete antibiotic utilization data were excluded, yielding a final study population of 2,138 children. Among these, blood samples were obtained from 2,134 (>99%) children for culture, pleural fluid from 87 (4%) children, bronchoalveolar lavage fluid from 31 (1%) children, and endotracheal aspirate from 80 (4%) children. Across all culture types, there were 2,332 initial cultures; 2,150 (92%) were collected within the first 24 hours.
Staphylococcal pneumonia was detected in 23 of the 2,138 children (1% [95% CI 0.7, 1.6]; 17 MRSA, 6 MSSA). Of these, 6/23 (26%) had bacteremia, 12/23 (52%) had a positive pleural fluid, and 9/23 (39%) had a positive culture from bronchoalveolar lavage fluid or endotracheal aspirate; 4/23 (17%) children had S. aureus detected from more than one site. Three children (13%) with S. aureus had a viral codetection, including two with influenza.
Compared with children with nonstaphylococcal pneumonia, those with staphylococcal pneumonia were more likely to have a parapneumonic effusion (78% vs 12%, P < .001), but less likely to have cough (78% vs 95%, P < .001). Other baseline characteristics were similar between the two groups. Children with staphylococcal pneumonia had more adverse outcomes than those without (Table), including longer median length of stay (10 vs 3 days, P < .001), more frequent admission to intensive care (83% vs 21%, P < .001), and more frequent invasive mechanical ventilation (65% vs 7%, P < .001). Similar findings were noted when staphylococcal pneumonia was compared with pneumonia caused due to other bacterial pathogens (n = 124). There were no significant differences in baseline characteristics or clinical course between children with MRSA and MSSA pneumonia, although the numbers were small. Overall, S. aureus was detected in 18/267 (7%) children with parapneumonic effusion and 19/462 (4%) children admitted to intensive care. Importantly, there were no confirmed S. aureus cases among children with less severe pneumonia, defined as lacking both parapneumonic effusion and intensive care admission (n = 1,488).
Overall, 519 children (24%) received antistaphylococcal therapy during their hospitalization (512/519, 99% received anti-MRSA therapy), including 22 of the 23 children with S. aureus detected (the only child without antistaphylococcal therapy had S. aureus detected from a high-quality endotracheal tube aspirate only and also had respiratory syncytial virus detected). Clindamycin was most often used (n = 266, 51%), followed by vancomycin (n = 128, 24%), clindamycin plus vancomycin (n = 83, 16%), and others (n = 42, 8%). During the first two days of hospitalization, 479 children (22%) received antistaphylococcal therapy (477 received anti-MRSA therapy). After the first two days, 351 children (16%) received antistaphylococcal therapy (346/351, 99% received anti-MRSA therapy). Use of antistaphylococcal therapy was very common in those admitted to intensive care (182/462, 39%; all but two received anti-MRSA therapy) and in those requiring invasive mechanical ventilation (103/159, 65%). Among those lacking both parapneumonic effusion and intensive care admission (n = 1488), 232 (16%) received antistaphylococcal therapy.
DISCUSSION
In our large, population-based study of >2,000 children hospitalized with community-acquired pneumonia, S. aureus was identified in only 1% of children. Compared with children with other pneumonia etiologies, staphylococcal pneumonia was associated with increased disease severity. Among the small numbers studied, no differences in outcomes were found between children with MRSA and MSSA disease. Despite the low prevalence of staphylococcal pneumonia, almost 1 in 4 children received antistaphylococcal antibiotic therapy; anti-MRSA therapy was used almost exclusively.
The severity of staphylococcal pneumonia was striking, with >80% of children with S. aureus detected being admitted to intensive care, about 65% requiring invasive mechanical ventilation, and >75% with parapneumonic effusion. These findings are similar to those of prior retrospective studies.4,10 The association between staphylococcal pneumonia and adverse outcomes underscores the importance of prompt institution of antimicrobial therapy targeting S. aureus in high-risk patients. This is noteworthy given recent epidemiological data demonstrating increases in MSSA relative to MRSA infections in children,6 and the known superiority of beta-lactam versus vancomycin for MSSA infections, including pneumonia.11
Although detection of staphylococcal infection was rare, almost a quarter of children received antistaphylococcal therapy; nearly all of these children received anti-MRSA therapy. Confirming a bacterial etiology of pneumonia, however, is challenging. Given the severity associated with staphylococcal pneumonia, it is not surprising that use of antistaphylococcal therapy outpaced staphylococcal detections. Antistaphylococcal therapy was especially common in those with severe pneumonia, suggesting that disease severity is an important factor that influences initial antibiotic treatment decisions. Even so, two children with MRSA detected did not initially receive anti-MRSA therapy, highlighting the challenge of balancing judicious antibiotic selection along with ensuring effective treatment. Perhaps more striking is the finding that 16% of children received antistaphylococcal therapy beyond the first two days of hospitalization, presumably after the initial culture results were available. This suggests that clinicians are reluctant to stop antistaphylococcal therapy when the etiology is unknown, although certain features, such as negative cultures, rapid clinical improvement, and lack of risk factors for staphylococcal disease, may provide important clues to support de-escalation of empiric antibiotic therapy. It is also possible that some antibiotics with antistaphylococcal activity were used for alternative indications (eg, clindamycin for penicillin allergy or concern for aspiration pneumonia).
A simple strategy for tailoring antibiotic treatment is maximizing opportunities to identify a causative pathogen. Despite the very low yield of blood cultures in children with pneumonia overall, bacteremia is more common in children with severe pneumonia and those with parapneumonic effusion, especially when cultures are obtained prior to antibiotic use.12,13 Similarly, obtaining pleural fluid is often therapeutic and significantly improves the chances of identifying a bacterial pathogen.14 Moreover, at least one study suggests that S. aureus is much less likely in cases of culture-negative parapneumonic effusions.15 Institutional guidelines, order sets, and antimicrobial stewardship teams are also effective strategies that can facilitate judicious antibiotic use. In particular, stewardship experts can be very useful in assisting clinicians around de-escalation of therapy.16 Use of procalcitonin, a biomarker associated with bacterial infections,17 and prognostic tools to identify risk for adverse outcomes,18 may also inform treatment decisions and are deserving of further study.
Our study must be considered in
Our study demonstrates a very low prevalence of S. aureus detection among children hospitalized with pneumonia and highlights the association between staphylococcal disease and adverse in-hospital outcomes. We also document important discrepancies between disease prevalence and utilization of antistaphylococcal therapy, especially anti-MRSA therapy. Improved approaches are needed to minimize overuse of antistaphylococcal antibiotics while also ensuring adequate therapy for those who need it.
Disclosures
Drs. Zhu, Edwards, Self, Ampofo, Arnold, McCullers, and Williams report grants from the Centers for Disease Control and Prevention during the conduct of the study. Ms. Frush has nothing to disclose. Dr. Jain has nothing to disclose. Dr. Grijalva reports other from Merck, grants and other from Sanofi, other from Pfizer, grants from CDC, grants from AHRQ, grants from NIH, and grants from Campbell Alliance, outside the submitted work. Dr. Self reports grants from CDC, during the conduct of the study; personal fees from Cempra Pharmaceuticals, grants and personal fees from Ferring Pharmaceuticals, personal fees from BioTest AG, personal fees from Abbott Point of Care, personal fees from Gilead Pharmaceuticals, personal fees from Pfizer, grants from Merck, outside the submitted work. Dr. Thomsen has nothing to disclose. Dr. Ampofo reports grants from CDC, during the conduct of the study; other from GlaxoSmithKline, other from Cubist Pharmaceuticals outside the submitted work; and KA collaborate with BioFire Diagnostics, Inc. (formerly Idaho Technology, Inc.) on several NIH grants. Dr. Pavia reports grants from NAID/NIH, grants from NAID/NIH, grants from CDC, personal fees from WebMD, personal fees from Antimicrobial Therapy Inc., outside the submitted work.
Funding
This work was supported by the National Institute of Allergy and Infectious Diseases of the National Institutes of Health under Award Number K23AI104779 to D.J.W. and Award 1K23AI113150 to I.P.T., the National Institute of General Medical Sciences under Award K23GM110469 to W.H.S., and the Agency for Healthcare Research and Quality under Award R03HS022342 to C.G.G. The EPIC study was supported by the Influenza Division in the National Center for Immunizations and Respiratory Diseases at the Centers for Disease Control and Prevention through cooperative agreements with each study site and was based on a competitive research funding opportunity. The findings and conclusions in this report are those of the authors and do not necessarily represent the views of the National Institute of Allergy and Infectious Diseases, the National Institute of General Medical Sciences, the Agency for Healthcare Research and Quality, or the Centers for Disease Control and Prevention.
1. Akil N, Muhlebach MS. Biology and management of methicillin resistant Staphylococcus aureus in cystic fibrosis. Pediatr Pulmonol. 2018. doi: 10.1002/ppul.24139. PubMed
2. Srinivasan R, Asselin J, Gildengorin G, Wiener-Kronish J, Flori HR. A prospective study of ventilator-associated pneumonia in children. Pediatrics.
2009;123(4):1108-1115. doi: 10.1542/peds.2008-1211. PubMed
3. Gonzalez BE, Martinez-Aguilar G, Hulten KG, et al. Severe Staphylococcal sepsis in adolescents in the era of community-acquired methicillin-resistant Staphylococcus aureus. Pediatrics. 2005;115(3):642-648. doi: 10.1542/peds.2004-2300. PubMed
4. Carrillo-Marquez MA, Hulten KG, Hammerman W, Lamberth L, Mason EO, Kaplan SL. Staphylococcus aureus pneumonia in children in the era of community-acquired methicillin-resistance at Texas Children’s Hospital. Pediatr Infect Dis J. 2011;30(7):545-550. doi: 10.1097/INF.0b013e31821618be. PubMed
5. Bradley JS, Byington CL, Shah SS, et al. The management of community-acquired pneumonia in infants and children older than 3 months of age: clinical practice guidelines by the pediatric infectious diseases society and the infectious diseases society of America. Clin Infect Dis. 2011;53(7):e25-e76. doi: 10.1093/cid/cir531. PubMed
6. Sutter DE, Milburn E, Chukwuma U, Dzialowy N, Maranich AM, Hospenthal DR. Changing susceptibility of Staphylococcus aureus in a US pediatric population. Pediatrics. 2016;137(4):e20153099–e20153099. doi: 10.1542/peds.2015-3099. PubMed
7. Sakoulas G, Moellering RC, Jr. Increasing antibiotic resistance among methicillin-resistant Staphylococcus aureus strains. Clin Infect Dis. 2008;46(Suppl 5):S360-S367. doi: 10.1086/533592. PubMed
8. Rubinstein E, Kollef MH, Nathwani D. Pneumonia caused by methicillin-resistant
Staphylococcus aureus. Clin Infect Dis. 2008;46(Suppl 5):S378-S385. doi: 10.1086/533594. PubMed
9. Jain S, Williams DJ, Arnold SR, et al. Community-acquired pneumonia requiring hospitalization among U.S. children. N Engl J Med. 2015;372(9):835-845. doi: 10.1056/NEJMoa1405870. PubMed
10. Kallen AJ, Reed C, Patton M, Arnold KE, Finelli L, Hageman J. Staphylococcus aureus community-onset pneumonia in patients admitted to children’s hospitals during autumn and winter of 2006-2007. Epidemiol Infect. 2010;138(5):666-672. doi: 10.1017/S095026880999135X. PubMed
11. González C, Rubio M, Romero-Vivas J, González M, Picazo JJ. Bacteremic pneumonia due to Staphylococcus aureus: A comparison of disease caused by methicillin-resistant and methicillin-susceptible organisms. Clin Infect Dis. 1999;29(5):1171-1177. doi: 10.1086/313440. PubMed
12. Myers AL, Hall M, Williams DJ, et al. Prevalence of bacteremia in hospitalized pediatric patients with community-acquired pneumonia. Pediatr Infect Dis J. 2013;32(7):736-740. doi: 10.1097/INF.0b013e318290bf63. PubMed
13. Iroh Tam PY, Bernstein E, Ma X, Ferrieri P. Blood culture in evaluation of pediatric community-acquired pneumonia: A systematic review and meta-analysis. Hosp Pediatr. 2015;5(6):324-336. doi: 10.1542/hpeds.2014-0138. PubMed
14. Byington CL, Spencer LY, Johnson TA, et al. An epidemiological investigation of a sustained high rate of pediatric parapneumonic empyema: risk factors and microbiological associations. Clin Infect Dis. 2002;34(4):434-440. doi: 10.1086/338460. PubMed
15. Blaschke AJ, Heyrend C, Byington CL, et al. Molecular analysis improves pathogen identifi cation and epidemiologic study of pediatric parapneumonic empyema. Pediatr Infect Dis J. 2011;30(4):289-294. doi: 10.1097/INF.0b013e3182002d14. PubMed
16. Banerjee R, Teng CB, Cunningham SA, et al. Randomized trial of rapid multiplex polymerase chain reaction-based blood culture identifi cation and susceptibility testing. Clin Infect Dis. 2015;61(7):1071-1080. doi: 10.1093/cid/civ447. PubMed
17. Stockmann C, Ampofo K, Killpack J, et al. Procalcitonin accurately identifies hospitalized children with low risk of bacterial community-acquired pneumonia. J Pediatr Infect Dis Soc. 2018;7(1):46–53. doi: 10.1093/jpids/piw091. PubMed
18. Williams DJ, Zhu Y, Grijalva CG, et al. Predicting severe pneumonia outcomes in children. Pediatrics. 2016;138(4). doi: 10.1542/peds.2016-1019. PubMed
19. Brogan TV, Hall M, Williams DJ, et al. Variability in processes of care and outcomes among children hospitalized with community-acquired pneumonia. Pediatr Infect Dis J. 2012;31(10):1036-1041. doi: 10.1097/INF.0b013e-31825f2b10. PubMed
1. Akil N, Muhlebach MS. Biology and management of methicillin resistant Staphylococcus aureus in cystic fibrosis. Pediatr Pulmonol. 2018. doi: 10.1002/ppul.24139. PubMed
2. Srinivasan R, Asselin J, Gildengorin G, Wiener-Kronish J, Flori HR. A prospective study of ventilator-associated pneumonia in children. Pediatrics.
2009;123(4):1108-1115. doi: 10.1542/peds.2008-1211. PubMed
3. Gonzalez BE, Martinez-Aguilar G, Hulten KG, et al. Severe Staphylococcal sepsis in adolescents in the era of community-acquired methicillin-resistant Staphylococcus aureus. Pediatrics. 2005;115(3):642-648. doi: 10.1542/peds.2004-2300. PubMed
4. Carrillo-Marquez MA, Hulten KG, Hammerman W, Lamberth L, Mason EO, Kaplan SL. Staphylococcus aureus pneumonia in children in the era of community-acquired methicillin-resistance at Texas Children’s Hospital. Pediatr Infect Dis J. 2011;30(7):545-550. doi: 10.1097/INF.0b013e31821618be. PubMed
5. Bradley JS, Byington CL, Shah SS, et al. The management of community-acquired pneumonia in infants and children older than 3 months of age: clinical practice guidelines by the pediatric infectious diseases society and the infectious diseases society of America. Clin Infect Dis. 2011;53(7):e25-e76. doi: 10.1093/cid/cir531. PubMed
6. Sutter DE, Milburn E, Chukwuma U, Dzialowy N, Maranich AM, Hospenthal DR. Changing susceptibility of Staphylococcus aureus in a US pediatric population. Pediatrics. 2016;137(4):e20153099–e20153099. doi: 10.1542/peds.2015-3099. PubMed
7. Sakoulas G, Moellering RC, Jr. Increasing antibiotic resistance among methicillin-resistant Staphylococcus aureus strains. Clin Infect Dis. 2008;46(Suppl 5):S360-S367. doi: 10.1086/533592. PubMed
8. Rubinstein E, Kollef MH, Nathwani D. Pneumonia caused by methicillin-resistant
Staphylococcus aureus. Clin Infect Dis. 2008;46(Suppl 5):S378-S385. doi: 10.1086/533594. PubMed
9. Jain S, Williams DJ, Arnold SR, et al. Community-acquired pneumonia requiring hospitalization among U.S. children. N Engl J Med. 2015;372(9):835-845. doi: 10.1056/NEJMoa1405870. PubMed
10. Kallen AJ, Reed C, Patton M, Arnold KE, Finelli L, Hageman J. Staphylococcus aureus community-onset pneumonia in patients admitted to children’s hospitals during autumn and winter of 2006-2007. Epidemiol Infect. 2010;138(5):666-672. doi: 10.1017/S095026880999135X. PubMed
11. González C, Rubio M, Romero-Vivas J, González M, Picazo JJ. Bacteremic pneumonia due to Staphylococcus aureus: A comparison of disease caused by methicillin-resistant and methicillin-susceptible organisms. Clin Infect Dis. 1999;29(5):1171-1177. doi: 10.1086/313440. PubMed
12. Myers AL, Hall M, Williams DJ, et al. Prevalence of bacteremia in hospitalized pediatric patients with community-acquired pneumonia. Pediatr Infect Dis J. 2013;32(7):736-740. doi: 10.1097/INF.0b013e318290bf63. PubMed
13. Iroh Tam PY, Bernstein E, Ma X, Ferrieri P. Blood culture in evaluation of pediatric community-acquired pneumonia: A systematic review and meta-analysis. Hosp Pediatr. 2015;5(6):324-336. doi: 10.1542/hpeds.2014-0138. PubMed
14. Byington CL, Spencer LY, Johnson TA, et al. An epidemiological investigation of a sustained high rate of pediatric parapneumonic empyema: risk factors and microbiological associations. Clin Infect Dis. 2002;34(4):434-440. doi: 10.1086/338460. PubMed
15. Blaschke AJ, Heyrend C, Byington CL, et al. Molecular analysis improves pathogen identifi cation and epidemiologic study of pediatric parapneumonic empyema. Pediatr Infect Dis J. 2011;30(4):289-294. doi: 10.1097/INF.0b013e3182002d14. PubMed
16. Banerjee R, Teng CB, Cunningham SA, et al. Randomized trial of rapid multiplex polymerase chain reaction-based blood culture identifi cation and susceptibility testing. Clin Infect Dis. 2015;61(7):1071-1080. doi: 10.1093/cid/civ447. PubMed
17. Stockmann C, Ampofo K, Killpack J, et al. Procalcitonin accurately identifies hospitalized children with low risk of bacterial community-acquired pneumonia. J Pediatr Infect Dis Soc. 2018;7(1):46–53. doi: 10.1093/jpids/piw091. PubMed
18. Williams DJ, Zhu Y, Grijalva CG, et al. Predicting severe pneumonia outcomes in children. Pediatrics. 2016;138(4). doi: 10.1542/peds.2016-1019. PubMed
19. Brogan TV, Hall M, Williams DJ, et al. Variability in processes of care and outcomes among children hospitalized with community-acquired pneumonia. Pediatr Infect Dis J. 2012;31(10):1036-1041. doi: 10.1097/INF.0b013e-31825f2b10. PubMed
©2018 Society of Hospital Medicine
TCS Among Children with Pneumonia
National guidelines for the management of childhood pneumonia highlight the need for the development of objective outcome measures to inform clinical decision making, establish benchmarks of care, and compare treatments and interventions.[1] Time to clinical stability (TCS) is a measure reported in adult pneumonia studies that incorporates vital signs, ability to eat, and mental status to objectively assess readiness for discharge.[2, 3, 4] TCS has not been validated among children as it has in adults,[5, 6, 7, 8] although such measures could prove useful for assessing discharge readiness with applications in both clinical and research settings. The objective of our study was to test the performance of pediatric TCS measures among children hospitalized with pneumonia.
METHODS
Study Population
We studied children hospitalized with community‐acquired pneumonia at Monroe Carell Jr. Children's Hospital at Vanderbilt between January 6, 2010 and May 9, 2011. Study children were enrolled as part of the Centers for Disease Control & Prevention (CDC) Etiology of Pneumonia in the Community (EPIC) study, a prospective, population‐based study of community‐acquired pneumonia hospitalizations. Detailed enrollment criteria for the EPIC study were reported previously.[9] Institutional review boards at Vanderbilt University and the CDC approved this study. Informed consent was obtained from enrolled families.
Data Elements and Study Definitions
Baseline data, including demographics, illness history, comorbidities, and clinical outcomes (eg, length of stay [LOS], intensive care admission), were systematically and prospectively collected. Additionally, data for 4 physiologic parameters, including temperature, heart rate, respiratory rate, and use of supplemental oxygen were obtained from the electronic medical record. These parameters were measured at least every 6 hours from admission through discharge as part of routine care. Readmissions within 7 calendar days of discharge were also obtained from the electronic medical record.
Stability for each parameter was defined as follows: normal temperature (36.037.9C), normal respiratory and heart rates in accordance with Pediatric Advanced Life Support age‐based values (see Supporting Table 1 in the online version of this article),[10] and no administration of supplemental oxygen. If the last recorded value for a given parameter was abnormal, that parameter was considered unstable at discharge. Otherwise, the time and date of the last abnormal value for each parameter was subtracted from admission time and date to determine TCS for that parameter in hours.
To determine overall stability, we evaluated 4 combination TCS measures, each incorporating 2 individual parameters. All combinations included respiratory rate and need for supplemental oxygen, as these parameters are the most explicit clinical indicators of pneumonia. Stability for each combination measure was defined as normalization of all included measures.
Clinical Outcomes for the Combined TCS Measures
The 4 combined TCS measures were compared against clinical outcomes including hospital LOS (measured in hours) and an ordinal severity scale. The ordinal scale categorized children into 3 mutually exclusive groups as follows: nonsevere (hospitalization without need for intensive care or empyema requiring drainage), severe (intensive care admission without invasive mechanical ventilation or vasopressor support and no empyema requiring drainage), and very severe (invasive mechanical ventilation, vasopressor support, or empyema requiring drainage).
Statistical Analysis
Categorical and continuous variables were summarized using frequencies and percentages and median and interquartile range (IQR) values, respectively. Analyses were stratified by age (<2 years, 24 years, 517 years). We also plotted summary statistics for the combined measures and LOS, and computed the median absolute difference between these measures for each level of the ordinal severity scale. Analyses were conducted using Stata 13 (StataCorp, College Station, TX).
RESULTS
Study Population
Among 336 children enrolled in the EPIC study at Vanderbilt during the study period, 334 (99.4%) with complete data were included. Median age was 33 months (IQR, 1480). Median LOS was 56.4 hours (IQR, 41.591.7). There were 249 (74.5%) children classified as nonsevere, 39 (11.7) as severe, and 46 (13.8) as very severe (for age‐based characteristics see Supporting Table 2 in the online version of this article). Overall, 12 (3.6%) children were readmitted within 7 days of discharge.
Individual Stability Parameters
Overall, 323 (96.7%) children had 1 parameter abnormal on admission. Respiratory rate (81.4%) was the most common abnormal parameter, followed by abnormal temperature (71.4%), use of supplemental oxygen (63.8%), and abnormal heart rate (54.4%). Overall, use of supplemental oxygen had the longest TCS, followed by respiratory rate (Table 1). In comparison, heart rate and temperature stabilized relatively quickly.
Parameter | <2 Years, n=130 | 24 Years, n=90 | 517 Years, n=101 | |||
---|---|---|---|---|---|---|
No. (%)* | Median (IQR) TCS, h | No. (%) | Median (IQR) TCS, h | No. (%) | Median (IQR) TCS, h | |
| ||||||
Respiratory rate | 97 (74.6) | 38.6 (18.768.9) | 63 (70.0) | 31.6 (9.561.9) | 63 (62.4) | 24.3 (10.859.2) |
Oxygen | 90 (69.2) | 39.5 (19.273.6) | 58 (64.4) | 44.2 (2477.6) | 61 (60.4) | 38.3 (1870.6) |
Heart rate | 21 (16.2) | 4.5 (0.318.4) | 73 (81.1) | 21.8 (5.751.9) | 62 (61.4) | 18 (5.842.2) |
Temperature | 101 (77.7) | 14.5 (4.545.3) | 61 (67.8) | 18.4 (2.842.8) | 62 (61.4) | 10.6 (0.834) |
Seventy children (21.0%) had 1 parameter abnormal at discharge, including abnormal respiratory rate in 13.7%, heart rate in 7.0%, and temperature in 3.3%. One child (0.3%) was discharged with supplemental oxygen. Ten children (3.0%) had 2 parameters abnormal at discharge. There was no difference in 7‐day readmissions for children with 1 parameter abnormal at discharge (1.4%) compared to those with no abnormal parameters at discharge (4.4%, P=0.253).
Combination TCS Measures
Within each age group, the percentage of children achieving stability was relatively consistent across the 4 combined TCS measures (Table 2); however, more children were considered unstable at discharge (and fewer classified as stable on admission) as the number of included parameters increased. More children <5 years of age reached stability (range, 80.0%85.6%) compared to children 5 years of age (range, 68.3%72.3%). We also noted increasing median TCS with increasing disease severity (Figure 1, P<0.01) (see Supporting Fig. 1AC in the online version of this article); TCS was only slightly shorter than LOS across all 3 levels of the severity scale.
TCS Measures | <2 Years, n=130 | 24 Years, n=90 | 517 Years, n=101 | P Value | |||
---|---|---|---|---|---|---|---|
No. (%)* | Median (IQR) TCS, h | No. (%) | Median (IQR) TCS, h | No. (%) | Median (IQR) TCS, h | ||
| |||||||
RR+O2 | 108 (83.1) | 40.5 (20.175.0) | 72 (80.0) | 39.6 (15.679.2) | 69 (68.3) | 30.4 (14.759.2) | 0.08 |
RR+O2+HR | 109 (83.8) | 40.2 (19.573.9) | 73 (81.1) | 35.9 (15.977.6) | 68 (67.3) | 29.8 (17.256.6) | 0.11 |
RR+O2+T | 110 (84.6) | 40.5 (20.770.1) | 77 (85.6) | 39.1 (18.477.6) | 73 (72.3) | 28.2 (14.744.7) | 0.03 |
RR+O2+HR+T | 110 (84.6) | 40.5 (20.770.1) | 72 (80.0) | 39.7 (20.177.5) | 71 (70.3) | 29.2 (18.254) | 0.05 |
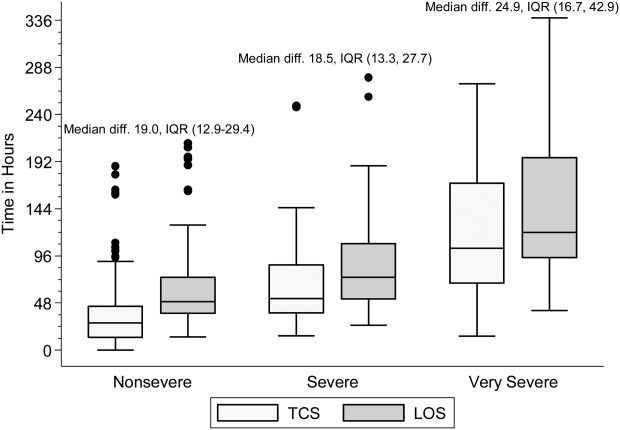
DISCUSSION
Our study demonstrates that longitudinal TCS measures consisting of routinely collected physiologic parameters may be useful for objectively assessing disease recovery and clinical readiness for discharge among children hospitalized with pneumonia. A simple TCS measure incorporating respiratory rate and oxygen requirement performed similarly to the more complex combinations and classified fewer children as unstable at discharge. However, we also note several challenges that deserve additional study prior to the application of a pediatric TCS measure in clinical and research settings.
Vital signs and supplemental oxygen use are used clinically to assess disease severity and response to therapy among children with acute respiratory illness. Because these objective parameters are routinely collected among hospitalized children, the systematization of these data could inform clinical decision making around hospital discharge. Similar to early warning scores used to detect impending clinical deterioration,[11] TCS measures, by signaling normalization of stability parameters in a consistent and objective manner, could serve as an early signal of readiness for discharge. However, maximizing the clinical utility of TCS would require embedding the process within the electronic health record, a tool that could also have implications for the Centers for Medicare and Medicaid Services' meaningful use regulations.[12]
TCS could also serve as an outcome measure in research and quality efforts. Increased disease severity was associated with longer TCS for the 4 combined measures; TCS also demonstrated strong agreement with LOS. Furthermore, TCS minimizes the influence of factors unrelated to disease that may impact LOS (eg, frequency of hospital rounds, transportation difficulties, or social impediments to discharge), an advantage when studying outcomes for research and quality benchmarking.
The percentage of children reaching stability and the median TCS for the combined measures demonstrated little variation within each age group, likely because respiratory rate and need for supplemental oxygen, 2 of the parameters with the longest individual time to stability, were also included in each of the combination measures. This suggests that less‐complex measures incorporating only respiratory rate and need for supplemental oxygen may be sufficient to assess clinical stability, particularly because these parameters are objectively measured and possess a direct physiological link to pneumonia. In contrast, the other parameters may be more often influenced by factors unrelated to disease severity.
Our study also highlights several shortcomings of the pediatric TCS measures. Despite use of published, age‐based reference values,[13] we noted wide variation in the achievement of stability across individual parameters, especially for children 5 years old. Overall, 21% of children had 1 abnormal parameter at discharge. Even the simplest combined measure classified 13.4% of children as unstable at discharge. Discharge with unstable parameters was not associated with 7‐day readmission, although our study was underpowered to detect small differences. Additional study is therefore needed to evaluate less restrictive cutoff values on calculated TCS and the impact of hospital discharge prior to reaching stability. In particular, relaxing the upper limit for normal respiratory rate in adolescents (16 breaths per minute) to more closely approximate the adult TCS parameter (24 breaths per minute) should be explored. Refinement and standardization of age‐based vital sign reference values specific to hospitalized children may also improve the performance of these measures.[14]
Several limitations deserve discussion. TCS parameters and readmission data were abstracted retrospectively from a single institution, and our findings may not be generalizable. Although clinical staff routinely measured these data, measurement variation likely exists. Nevertheless, such variation is likely systematic, limiting the impact of potential misclassification. TCS was calculated based on the last abnormal value for each parameter; prior fluctuations between normal and abnormal periods of stability were not captured. We were unable to assess room air oxygen saturations. Instead, supplemental oxygen use served as a surrogate for hypoxia. At our institution, oxygen therapy is provided for children with pneumonia to maintain oxygen saturations of 90% to 92%. We did not assess work of breathing (a marker of severe pneumonia) or ability to eat (a component of adult TCS measures). We initially considered the evaluation of intravenous fluids as a proxy for ability to eat (addition of this parameter to the 4 parameter TCS resulted in a modest increase in median time to stability, data not shown); however, we felt the lack of institutional policy and subjective nature of this parameter detracted from our study's objectives. Finally, we were not able to determine clinical readiness for discharge beyond the measurement of vital sign parameters. Therefore, prospective evaluation of the proposed pediatric TCS measures in broader populations will be important to build upon our findings, refine stability parameters, and test the utility of new parameters (eg, ability to eat, work of breathing) prior to use in clinical settings.
Our study provides an initial evaluation of TCS measures for assessing severity and recovery among children hospitalized with pneumonia. Similar to adults, such validated TCS measures may ultimately prove useful for improving the quality of both clinical care and research, although additional study to more clearly define stability criteria is needed prior to implementation.
Disclosures
This work was supported by the National Institute of Allergy and Infectious Diseases of the National Institutes of Health under Award Number K23AI104779 to Dr. Williams. The EPIC study was supported by the Influenza Division in the National Center for Immunizations and Respiratory Diseases at the Centers for Disease Control and Prevention through cooperative agreements with each study site and was based on a competitive research funding opportunity. The findings and conclusions in this report are those of the authors and do not necessarily represent the views of the Centers for Disease Control and Prevention or the National Institutes of Health. Dr. Grijalva serves as a consultant to Glaxo‐Smith‐Kline and Pfizer outside of the scope of this article. Dr. Edwards is supported through grants from Novartis for the conduction of a Group B strep vaccine study and serves as the Chair of the Data Safety and Monitoring Data Committee for Influenza Study outside the scope of this article. Dr. Self reports grants from CareFusion, BioMerieux, Affinium Pharmaceuticals, Astute Medical, Crucell Holland BV, BRAHMS GmbH, Pfizer, Rapid Pathogen Screening, Venaxis, BioAegis Inc., Sphingotec GmbH, and Cempra Pharmaceuticals; personal fees from BioFire Diagnostics and Venaxis, Inc; and patent 13/632,874 (Sterile Blood Culture Collection System) pending; all outside the scope of this article.
- Healthcare Cost and Utilization Project. Available at: http://www.ahrq.gov/research/data/hcup/index.html. Accessed February 1, 2014.
- Time to clinical stability in patients hospitalized with community‐acquired pneumonia: implications for practice guidelines. JAMA. 1998;279:1452–1457. , , , et al.
- Neumofail Group. Reaching stability in community‐acquired pneumonia: the effects of the severity of disease, treatment, and the characteristics of patients. Clin Infect Dis. 2004;39:1783–1790. , , , et al.;
- Community‐Acquired Pneumonia Organization. The pneumonia severity index predicts time to clinical stability in patients with community‐acquired pneumonia. Int J Tuberc Lung Dis. 2006;10:739–743. , , , et al.;
- Efficacy of corticosteroids in community‐acquired pneumonia: a randomized double‐blinded clinical trial. Am J Respir Crit Care Med. 2010;181:975–982. , , , , .
- Early administration of antibiotics does not shorten time to clinical stability in patients with moderate‐to‐severe community‐acquired pneumonia. Chest 2003;124:1798–1804. , , , et al.
- A comparison between time to clinical stability in community‐acquired aspiration pneumonia and community‐acquired pneumonia. Intern Emerg Med. 2014;9:143–150. , , , .
- Community‐Acquired Pneumonia Organization (CAPO) Investigators. A worldwide perspective of atypical pathogens in community‐acquired pneumonia. Am J Respir Crit Care Med. 2007;175:1086–1093. , , , et al.;
- CDC EPIC Study Team. Community‐acquired pneumonia requiring hospitalization among U.S. children. N Engl J Med. 2015;372:835–845. , , , et al.;
- American Heart Association. 2005 American Heart Association (AHA) guidelines for cardiopulmonary resuscitation (CPR) and emergency cardiovascular care (ECC) of pediatric and neonatal patients: pediatric basic life support. Pediatrics. 2006;117:e989–e1004.
- Development and initial validation of the Bedside Paediatric Early Warning System score. Crit Care. 2009;13:R135. , , .
- Centers for Medicare and Medicaid Services. Regulations and guidance. EHR incentive programs. Available at: http://www.cms.gov/Regulations‐and‐Guidance/Legislation/EHRIncentivePrograms/index.html. Accessed February 20, 2015
- Development of heart and respiratory rate percentile curves for hospitalized children. Pediatrics. 2013;131:e1150–e1157. , , , , , .
- Length of stay after reaching clinical stability drives hospital costs associated with adult community‐acquired pneumonia. Scand J Infect Dis. 2013;45:219–226. , , , et al.
National guidelines for the management of childhood pneumonia highlight the need for the development of objective outcome measures to inform clinical decision making, establish benchmarks of care, and compare treatments and interventions.[1] Time to clinical stability (TCS) is a measure reported in adult pneumonia studies that incorporates vital signs, ability to eat, and mental status to objectively assess readiness for discharge.[2, 3, 4] TCS has not been validated among children as it has in adults,[5, 6, 7, 8] although such measures could prove useful for assessing discharge readiness with applications in both clinical and research settings. The objective of our study was to test the performance of pediatric TCS measures among children hospitalized with pneumonia.
METHODS
Study Population
We studied children hospitalized with community‐acquired pneumonia at Monroe Carell Jr. Children's Hospital at Vanderbilt between January 6, 2010 and May 9, 2011. Study children were enrolled as part of the Centers for Disease Control & Prevention (CDC) Etiology of Pneumonia in the Community (EPIC) study, a prospective, population‐based study of community‐acquired pneumonia hospitalizations. Detailed enrollment criteria for the EPIC study were reported previously.[9] Institutional review boards at Vanderbilt University and the CDC approved this study. Informed consent was obtained from enrolled families.
Data Elements and Study Definitions
Baseline data, including demographics, illness history, comorbidities, and clinical outcomes (eg, length of stay [LOS], intensive care admission), were systematically and prospectively collected. Additionally, data for 4 physiologic parameters, including temperature, heart rate, respiratory rate, and use of supplemental oxygen were obtained from the electronic medical record. These parameters were measured at least every 6 hours from admission through discharge as part of routine care. Readmissions within 7 calendar days of discharge were also obtained from the electronic medical record.
Stability for each parameter was defined as follows: normal temperature (36.037.9C), normal respiratory and heart rates in accordance with Pediatric Advanced Life Support age‐based values (see Supporting Table 1 in the online version of this article),[10] and no administration of supplemental oxygen. If the last recorded value for a given parameter was abnormal, that parameter was considered unstable at discharge. Otherwise, the time and date of the last abnormal value for each parameter was subtracted from admission time and date to determine TCS for that parameter in hours.
To determine overall stability, we evaluated 4 combination TCS measures, each incorporating 2 individual parameters. All combinations included respiratory rate and need for supplemental oxygen, as these parameters are the most explicit clinical indicators of pneumonia. Stability for each combination measure was defined as normalization of all included measures.
Clinical Outcomes for the Combined TCS Measures
The 4 combined TCS measures were compared against clinical outcomes including hospital LOS (measured in hours) and an ordinal severity scale. The ordinal scale categorized children into 3 mutually exclusive groups as follows: nonsevere (hospitalization without need for intensive care or empyema requiring drainage), severe (intensive care admission without invasive mechanical ventilation or vasopressor support and no empyema requiring drainage), and very severe (invasive mechanical ventilation, vasopressor support, or empyema requiring drainage).
Statistical Analysis
Categorical and continuous variables were summarized using frequencies and percentages and median and interquartile range (IQR) values, respectively. Analyses were stratified by age (<2 years, 24 years, 517 years). We also plotted summary statistics for the combined measures and LOS, and computed the median absolute difference between these measures for each level of the ordinal severity scale. Analyses were conducted using Stata 13 (StataCorp, College Station, TX).
RESULTS
Study Population
Among 336 children enrolled in the EPIC study at Vanderbilt during the study period, 334 (99.4%) with complete data were included. Median age was 33 months (IQR, 1480). Median LOS was 56.4 hours (IQR, 41.591.7). There were 249 (74.5%) children classified as nonsevere, 39 (11.7) as severe, and 46 (13.8) as very severe (for age‐based characteristics see Supporting Table 2 in the online version of this article). Overall, 12 (3.6%) children were readmitted within 7 days of discharge.
Individual Stability Parameters
Overall, 323 (96.7%) children had 1 parameter abnormal on admission. Respiratory rate (81.4%) was the most common abnormal parameter, followed by abnormal temperature (71.4%), use of supplemental oxygen (63.8%), and abnormal heart rate (54.4%). Overall, use of supplemental oxygen had the longest TCS, followed by respiratory rate (Table 1). In comparison, heart rate and temperature stabilized relatively quickly.
Parameter | <2 Years, n=130 | 24 Years, n=90 | 517 Years, n=101 | |||
---|---|---|---|---|---|---|
No. (%)* | Median (IQR) TCS, h | No. (%) | Median (IQR) TCS, h | No. (%) | Median (IQR) TCS, h | |
| ||||||
Respiratory rate | 97 (74.6) | 38.6 (18.768.9) | 63 (70.0) | 31.6 (9.561.9) | 63 (62.4) | 24.3 (10.859.2) |
Oxygen | 90 (69.2) | 39.5 (19.273.6) | 58 (64.4) | 44.2 (2477.6) | 61 (60.4) | 38.3 (1870.6) |
Heart rate | 21 (16.2) | 4.5 (0.318.4) | 73 (81.1) | 21.8 (5.751.9) | 62 (61.4) | 18 (5.842.2) |
Temperature | 101 (77.7) | 14.5 (4.545.3) | 61 (67.8) | 18.4 (2.842.8) | 62 (61.4) | 10.6 (0.834) |
Seventy children (21.0%) had 1 parameter abnormal at discharge, including abnormal respiratory rate in 13.7%, heart rate in 7.0%, and temperature in 3.3%. One child (0.3%) was discharged with supplemental oxygen. Ten children (3.0%) had 2 parameters abnormal at discharge. There was no difference in 7‐day readmissions for children with 1 parameter abnormal at discharge (1.4%) compared to those with no abnormal parameters at discharge (4.4%, P=0.253).
Combination TCS Measures
Within each age group, the percentage of children achieving stability was relatively consistent across the 4 combined TCS measures (Table 2); however, more children were considered unstable at discharge (and fewer classified as stable on admission) as the number of included parameters increased. More children <5 years of age reached stability (range, 80.0%85.6%) compared to children 5 years of age (range, 68.3%72.3%). We also noted increasing median TCS with increasing disease severity (Figure 1, P<0.01) (see Supporting Fig. 1AC in the online version of this article); TCS was only slightly shorter than LOS across all 3 levels of the severity scale.
TCS Measures | <2 Years, n=130 | 24 Years, n=90 | 517 Years, n=101 | P Value | |||
---|---|---|---|---|---|---|---|
No. (%)* | Median (IQR) TCS, h | No. (%) | Median (IQR) TCS, h | No. (%) | Median (IQR) TCS, h | ||
| |||||||
RR+O2 | 108 (83.1) | 40.5 (20.175.0) | 72 (80.0) | 39.6 (15.679.2) | 69 (68.3) | 30.4 (14.759.2) | 0.08 |
RR+O2+HR | 109 (83.8) | 40.2 (19.573.9) | 73 (81.1) | 35.9 (15.977.6) | 68 (67.3) | 29.8 (17.256.6) | 0.11 |
RR+O2+T | 110 (84.6) | 40.5 (20.770.1) | 77 (85.6) | 39.1 (18.477.6) | 73 (72.3) | 28.2 (14.744.7) | 0.03 |
RR+O2+HR+T | 110 (84.6) | 40.5 (20.770.1) | 72 (80.0) | 39.7 (20.177.5) | 71 (70.3) | 29.2 (18.254) | 0.05 |
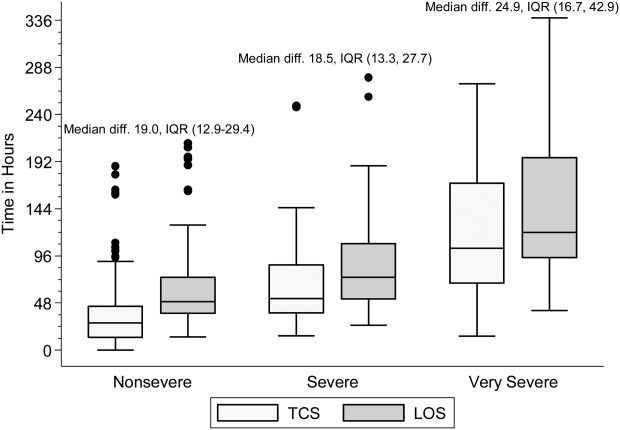
DISCUSSION
Our study demonstrates that longitudinal TCS measures consisting of routinely collected physiologic parameters may be useful for objectively assessing disease recovery and clinical readiness for discharge among children hospitalized with pneumonia. A simple TCS measure incorporating respiratory rate and oxygen requirement performed similarly to the more complex combinations and classified fewer children as unstable at discharge. However, we also note several challenges that deserve additional study prior to the application of a pediatric TCS measure in clinical and research settings.
Vital signs and supplemental oxygen use are used clinically to assess disease severity and response to therapy among children with acute respiratory illness. Because these objective parameters are routinely collected among hospitalized children, the systematization of these data could inform clinical decision making around hospital discharge. Similar to early warning scores used to detect impending clinical deterioration,[11] TCS measures, by signaling normalization of stability parameters in a consistent and objective manner, could serve as an early signal of readiness for discharge. However, maximizing the clinical utility of TCS would require embedding the process within the electronic health record, a tool that could also have implications for the Centers for Medicare and Medicaid Services' meaningful use regulations.[12]
TCS could also serve as an outcome measure in research and quality efforts. Increased disease severity was associated with longer TCS for the 4 combined measures; TCS also demonstrated strong agreement with LOS. Furthermore, TCS minimizes the influence of factors unrelated to disease that may impact LOS (eg, frequency of hospital rounds, transportation difficulties, or social impediments to discharge), an advantage when studying outcomes for research and quality benchmarking.
The percentage of children reaching stability and the median TCS for the combined measures demonstrated little variation within each age group, likely because respiratory rate and need for supplemental oxygen, 2 of the parameters with the longest individual time to stability, were also included in each of the combination measures. This suggests that less‐complex measures incorporating only respiratory rate and need for supplemental oxygen may be sufficient to assess clinical stability, particularly because these parameters are objectively measured and possess a direct physiological link to pneumonia. In contrast, the other parameters may be more often influenced by factors unrelated to disease severity.
Our study also highlights several shortcomings of the pediatric TCS measures. Despite use of published, age‐based reference values,[13] we noted wide variation in the achievement of stability across individual parameters, especially for children 5 years old. Overall, 21% of children had 1 abnormal parameter at discharge. Even the simplest combined measure classified 13.4% of children as unstable at discharge. Discharge with unstable parameters was not associated with 7‐day readmission, although our study was underpowered to detect small differences. Additional study is therefore needed to evaluate less restrictive cutoff values on calculated TCS and the impact of hospital discharge prior to reaching stability. In particular, relaxing the upper limit for normal respiratory rate in adolescents (16 breaths per minute) to more closely approximate the adult TCS parameter (24 breaths per minute) should be explored. Refinement and standardization of age‐based vital sign reference values specific to hospitalized children may also improve the performance of these measures.[14]
Several limitations deserve discussion. TCS parameters and readmission data were abstracted retrospectively from a single institution, and our findings may not be generalizable. Although clinical staff routinely measured these data, measurement variation likely exists. Nevertheless, such variation is likely systematic, limiting the impact of potential misclassification. TCS was calculated based on the last abnormal value for each parameter; prior fluctuations between normal and abnormal periods of stability were not captured. We were unable to assess room air oxygen saturations. Instead, supplemental oxygen use served as a surrogate for hypoxia. At our institution, oxygen therapy is provided for children with pneumonia to maintain oxygen saturations of 90% to 92%. We did not assess work of breathing (a marker of severe pneumonia) or ability to eat (a component of adult TCS measures). We initially considered the evaluation of intravenous fluids as a proxy for ability to eat (addition of this parameter to the 4 parameter TCS resulted in a modest increase in median time to stability, data not shown); however, we felt the lack of institutional policy and subjective nature of this parameter detracted from our study's objectives. Finally, we were not able to determine clinical readiness for discharge beyond the measurement of vital sign parameters. Therefore, prospective evaluation of the proposed pediatric TCS measures in broader populations will be important to build upon our findings, refine stability parameters, and test the utility of new parameters (eg, ability to eat, work of breathing) prior to use in clinical settings.
Our study provides an initial evaluation of TCS measures for assessing severity and recovery among children hospitalized with pneumonia. Similar to adults, such validated TCS measures may ultimately prove useful for improving the quality of both clinical care and research, although additional study to more clearly define stability criteria is needed prior to implementation.
Disclosures
This work was supported by the National Institute of Allergy and Infectious Diseases of the National Institutes of Health under Award Number K23AI104779 to Dr. Williams. The EPIC study was supported by the Influenza Division in the National Center for Immunizations and Respiratory Diseases at the Centers for Disease Control and Prevention through cooperative agreements with each study site and was based on a competitive research funding opportunity. The findings and conclusions in this report are those of the authors and do not necessarily represent the views of the Centers for Disease Control and Prevention or the National Institutes of Health. Dr. Grijalva serves as a consultant to Glaxo‐Smith‐Kline and Pfizer outside of the scope of this article. Dr. Edwards is supported through grants from Novartis for the conduction of a Group B strep vaccine study and serves as the Chair of the Data Safety and Monitoring Data Committee for Influenza Study outside the scope of this article. Dr. Self reports grants from CareFusion, BioMerieux, Affinium Pharmaceuticals, Astute Medical, Crucell Holland BV, BRAHMS GmbH, Pfizer, Rapid Pathogen Screening, Venaxis, BioAegis Inc., Sphingotec GmbH, and Cempra Pharmaceuticals; personal fees from BioFire Diagnostics and Venaxis, Inc; and patent 13/632,874 (Sterile Blood Culture Collection System) pending; all outside the scope of this article.
National guidelines for the management of childhood pneumonia highlight the need for the development of objective outcome measures to inform clinical decision making, establish benchmarks of care, and compare treatments and interventions.[1] Time to clinical stability (TCS) is a measure reported in adult pneumonia studies that incorporates vital signs, ability to eat, and mental status to objectively assess readiness for discharge.[2, 3, 4] TCS has not been validated among children as it has in adults,[5, 6, 7, 8] although such measures could prove useful for assessing discharge readiness with applications in both clinical and research settings. The objective of our study was to test the performance of pediatric TCS measures among children hospitalized with pneumonia.
METHODS
Study Population
We studied children hospitalized with community‐acquired pneumonia at Monroe Carell Jr. Children's Hospital at Vanderbilt between January 6, 2010 and May 9, 2011. Study children were enrolled as part of the Centers for Disease Control & Prevention (CDC) Etiology of Pneumonia in the Community (EPIC) study, a prospective, population‐based study of community‐acquired pneumonia hospitalizations. Detailed enrollment criteria for the EPIC study were reported previously.[9] Institutional review boards at Vanderbilt University and the CDC approved this study. Informed consent was obtained from enrolled families.
Data Elements and Study Definitions
Baseline data, including demographics, illness history, comorbidities, and clinical outcomes (eg, length of stay [LOS], intensive care admission), were systematically and prospectively collected. Additionally, data for 4 physiologic parameters, including temperature, heart rate, respiratory rate, and use of supplemental oxygen were obtained from the electronic medical record. These parameters were measured at least every 6 hours from admission through discharge as part of routine care. Readmissions within 7 calendar days of discharge were also obtained from the electronic medical record.
Stability for each parameter was defined as follows: normal temperature (36.037.9C), normal respiratory and heart rates in accordance with Pediatric Advanced Life Support age‐based values (see Supporting Table 1 in the online version of this article),[10] and no administration of supplemental oxygen. If the last recorded value for a given parameter was abnormal, that parameter was considered unstable at discharge. Otherwise, the time and date of the last abnormal value for each parameter was subtracted from admission time and date to determine TCS for that parameter in hours.
To determine overall stability, we evaluated 4 combination TCS measures, each incorporating 2 individual parameters. All combinations included respiratory rate and need for supplemental oxygen, as these parameters are the most explicit clinical indicators of pneumonia. Stability for each combination measure was defined as normalization of all included measures.
Clinical Outcomes for the Combined TCS Measures
The 4 combined TCS measures were compared against clinical outcomes including hospital LOS (measured in hours) and an ordinal severity scale. The ordinal scale categorized children into 3 mutually exclusive groups as follows: nonsevere (hospitalization without need for intensive care or empyema requiring drainage), severe (intensive care admission without invasive mechanical ventilation or vasopressor support and no empyema requiring drainage), and very severe (invasive mechanical ventilation, vasopressor support, or empyema requiring drainage).
Statistical Analysis
Categorical and continuous variables were summarized using frequencies and percentages and median and interquartile range (IQR) values, respectively. Analyses were stratified by age (<2 years, 24 years, 517 years). We also plotted summary statistics for the combined measures and LOS, and computed the median absolute difference between these measures for each level of the ordinal severity scale. Analyses were conducted using Stata 13 (StataCorp, College Station, TX).
RESULTS
Study Population
Among 336 children enrolled in the EPIC study at Vanderbilt during the study period, 334 (99.4%) with complete data were included. Median age was 33 months (IQR, 1480). Median LOS was 56.4 hours (IQR, 41.591.7). There were 249 (74.5%) children classified as nonsevere, 39 (11.7) as severe, and 46 (13.8) as very severe (for age‐based characteristics see Supporting Table 2 in the online version of this article). Overall, 12 (3.6%) children were readmitted within 7 days of discharge.
Individual Stability Parameters
Overall, 323 (96.7%) children had 1 parameter abnormal on admission. Respiratory rate (81.4%) was the most common abnormal parameter, followed by abnormal temperature (71.4%), use of supplemental oxygen (63.8%), and abnormal heart rate (54.4%). Overall, use of supplemental oxygen had the longest TCS, followed by respiratory rate (Table 1). In comparison, heart rate and temperature stabilized relatively quickly.
Parameter | <2 Years, n=130 | 24 Years, n=90 | 517 Years, n=101 | |||
---|---|---|---|---|---|---|
No. (%)* | Median (IQR) TCS, h | No. (%) | Median (IQR) TCS, h | No. (%) | Median (IQR) TCS, h | |
| ||||||
Respiratory rate | 97 (74.6) | 38.6 (18.768.9) | 63 (70.0) | 31.6 (9.561.9) | 63 (62.4) | 24.3 (10.859.2) |
Oxygen | 90 (69.2) | 39.5 (19.273.6) | 58 (64.4) | 44.2 (2477.6) | 61 (60.4) | 38.3 (1870.6) |
Heart rate | 21 (16.2) | 4.5 (0.318.4) | 73 (81.1) | 21.8 (5.751.9) | 62 (61.4) | 18 (5.842.2) |
Temperature | 101 (77.7) | 14.5 (4.545.3) | 61 (67.8) | 18.4 (2.842.8) | 62 (61.4) | 10.6 (0.834) |
Seventy children (21.0%) had 1 parameter abnormal at discharge, including abnormal respiratory rate in 13.7%, heart rate in 7.0%, and temperature in 3.3%. One child (0.3%) was discharged with supplemental oxygen. Ten children (3.0%) had 2 parameters abnormal at discharge. There was no difference in 7‐day readmissions for children with 1 parameter abnormal at discharge (1.4%) compared to those with no abnormal parameters at discharge (4.4%, P=0.253).
Combination TCS Measures
Within each age group, the percentage of children achieving stability was relatively consistent across the 4 combined TCS measures (Table 2); however, more children were considered unstable at discharge (and fewer classified as stable on admission) as the number of included parameters increased. More children <5 years of age reached stability (range, 80.0%85.6%) compared to children 5 years of age (range, 68.3%72.3%). We also noted increasing median TCS with increasing disease severity (Figure 1, P<0.01) (see Supporting Fig. 1AC in the online version of this article); TCS was only slightly shorter than LOS across all 3 levels of the severity scale.
TCS Measures | <2 Years, n=130 | 24 Years, n=90 | 517 Years, n=101 | P Value | |||
---|---|---|---|---|---|---|---|
No. (%)* | Median (IQR) TCS, h | No. (%) | Median (IQR) TCS, h | No. (%) | Median (IQR) TCS, h | ||
| |||||||
RR+O2 | 108 (83.1) | 40.5 (20.175.0) | 72 (80.0) | 39.6 (15.679.2) | 69 (68.3) | 30.4 (14.759.2) | 0.08 |
RR+O2+HR | 109 (83.8) | 40.2 (19.573.9) | 73 (81.1) | 35.9 (15.977.6) | 68 (67.3) | 29.8 (17.256.6) | 0.11 |
RR+O2+T | 110 (84.6) | 40.5 (20.770.1) | 77 (85.6) | 39.1 (18.477.6) | 73 (72.3) | 28.2 (14.744.7) | 0.03 |
RR+O2+HR+T | 110 (84.6) | 40.5 (20.770.1) | 72 (80.0) | 39.7 (20.177.5) | 71 (70.3) | 29.2 (18.254) | 0.05 |
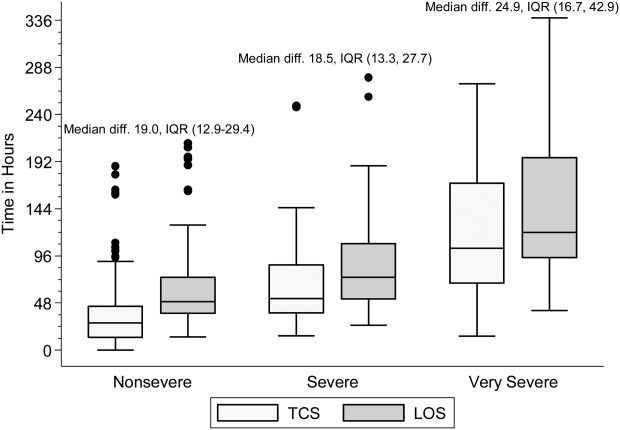
DISCUSSION
Our study demonstrates that longitudinal TCS measures consisting of routinely collected physiologic parameters may be useful for objectively assessing disease recovery and clinical readiness for discharge among children hospitalized with pneumonia. A simple TCS measure incorporating respiratory rate and oxygen requirement performed similarly to the more complex combinations and classified fewer children as unstable at discharge. However, we also note several challenges that deserve additional study prior to the application of a pediatric TCS measure in clinical and research settings.
Vital signs and supplemental oxygen use are used clinically to assess disease severity and response to therapy among children with acute respiratory illness. Because these objective parameters are routinely collected among hospitalized children, the systematization of these data could inform clinical decision making around hospital discharge. Similar to early warning scores used to detect impending clinical deterioration,[11] TCS measures, by signaling normalization of stability parameters in a consistent and objective manner, could serve as an early signal of readiness for discharge. However, maximizing the clinical utility of TCS would require embedding the process within the electronic health record, a tool that could also have implications for the Centers for Medicare and Medicaid Services' meaningful use regulations.[12]
TCS could also serve as an outcome measure in research and quality efforts. Increased disease severity was associated with longer TCS for the 4 combined measures; TCS also demonstrated strong agreement with LOS. Furthermore, TCS minimizes the influence of factors unrelated to disease that may impact LOS (eg, frequency of hospital rounds, transportation difficulties, or social impediments to discharge), an advantage when studying outcomes for research and quality benchmarking.
The percentage of children reaching stability and the median TCS for the combined measures demonstrated little variation within each age group, likely because respiratory rate and need for supplemental oxygen, 2 of the parameters with the longest individual time to stability, were also included in each of the combination measures. This suggests that less‐complex measures incorporating only respiratory rate and need for supplemental oxygen may be sufficient to assess clinical stability, particularly because these parameters are objectively measured and possess a direct physiological link to pneumonia. In contrast, the other parameters may be more often influenced by factors unrelated to disease severity.
Our study also highlights several shortcomings of the pediatric TCS measures. Despite use of published, age‐based reference values,[13] we noted wide variation in the achievement of stability across individual parameters, especially for children 5 years old. Overall, 21% of children had 1 abnormal parameter at discharge. Even the simplest combined measure classified 13.4% of children as unstable at discharge. Discharge with unstable parameters was not associated with 7‐day readmission, although our study was underpowered to detect small differences. Additional study is therefore needed to evaluate less restrictive cutoff values on calculated TCS and the impact of hospital discharge prior to reaching stability. In particular, relaxing the upper limit for normal respiratory rate in adolescents (16 breaths per minute) to more closely approximate the adult TCS parameter (24 breaths per minute) should be explored. Refinement and standardization of age‐based vital sign reference values specific to hospitalized children may also improve the performance of these measures.[14]
Several limitations deserve discussion. TCS parameters and readmission data were abstracted retrospectively from a single institution, and our findings may not be generalizable. Although clinical staff routinely measured these data, measurement variation likely exists. Nevertheless, such variation is likely systematic, limiting the impact of potential misclassification. TCS was calculated based on the last abnormal value for each parameter; prior fluctuations between normal and abnormal periods of stability were not captured. We were unable to assess room air oxygen saturations. Instead, supplemental oxygen use served as a surrogate for hypoxia. At our institution, oxygen therapy is provided for children with pneumonia to maintain oxygen saturations of 90% to 92%. We did not assess work of breathing (a marker of severe pneumonia) or ability to eat (a component of adult TCS measures). We initially considered the evaluation of intravenous fluids as a proxy for ability to eat (addition of this parameter to the 4 parameter TCS resulted in a modest increase in median time to stability, data not shown); however, we felt the lack of institutional policy and subjective nature of this parameter detracted from our study's objectives. Finally, we were not able to determine clinical readiness for discharge beyond the measurement of vital sign parameters. Therefore, prospective evaluation of the proposed pediatric TCS measures in broader populations will be important to build upon our findings, refine stability parameters, and test the utility of new parameters (eg, ability to eat, work of breathing) prior to use in clinical settings.
Our study provides an initial evaluation of TCS measures for assessing severity and recovery among children hospitalized with pneumonia. Similar to adults, such validated TCS measures may ultimately prove useful for improving the quality of both clinical care and research, although additional study to more clearly define stability criteria is needed prior to implementation.
Disclosures
This work was supported by the National Institute of Allergy and Infectious Diseases of the National Institutes of Health under Award Number K23AI104779 to Dr. Williams. The EPIC study was supported by the Influenza Division in the National Center for Immunizations and Respiratory Diseases at the Centers for Disease Control and Prevention through cooperative agreements with each study site and was based on a competitive research funding opportunity. The findings and conclusions in this report are those of the authors and do not necessarily represent the views of the Centers for Disease Control and Prevention or the National Institutes of Health. Dr. Grijalva serves as a consultant to Glaxo‐Smith‐Kline and Pfizer outside of the scope of this article. Dr. Edwards is supported through grants from Novartis for the conduction of a Group B strep vaccine study and serves as the Chair of the Data Safety and Monitoring Data Committee for Influenza Study outside the scope of this article. Dr. Self reports grants from CareFusion, BioMerieux, Affinium Pharmaceuticals, Astute Medical, Crucell Holland BV, BRAHMS GmbH, Pfizer, Rapid Pathogen Screening, Venaxis, BioAegis Inc., Sphingotec GmbH, and Cempra Pharmaceuticals; personal fees from BioFire Diagnostics and Venaxis, Inc; and patent 13/632,874 (Sterile Blood Culture Collection System) pending; all outside the scope of this article.
- Healthcare Cost and Utilization Project. Available at: http://www.ahrq.gov/research/data/hcup/index.html. Accessed February 1, 2014.
- Time to clinical stability in patients hospitalized with community‐acquired pneumonia: implications for practice guidelines. JAMA. 1998;279:1452–1457. , , , et al.
- Neumofail Group. Reaching stability in community‐acquired pneumonia: the effects of the severity of disease, treatment, and the characteristics of patients. Clin Infect Dis. 2004;39:1783–1790. , , , et al.;
- Community‐Acquired Pneumonia Organization. The pneumonia severity index predicts time to clinical stability in patients with community‐acquired pneumonia. Int J Tuberc Lung Dis. 2006;10:739–743. , , , et al.;
- Efficacy of corticosteroids in community‐acquired pneumonia: a randomized double‐blinded clinical trial. Am J Respir Crit Care Med. 2010;181:975–982. , , , , .
- Early administration of antibiotics does not shorten time to clinical stability in patients with moderate‐to‐severe community‐acquired pneumonia. Chest 2003;124:1798–1804. , , , et al.
- A comparison between time to clinical stability in community‐acquired aspiration pneumonia and community‐acquired pneumonia. Intern Emerg Med. 2014;9:143–150. , , , .
- Community‐Acquired Pneumonia Organization (CAPO) Investigators. A worldwide perspective of atypical pathogens in community‐acquired pneumonia. Am J Respir Crit Care Med. 2007;175:1086–1093. , , , et al.;
- CDC EPIC Study Team. Community‐acquired pneumonia requiring hospitalization among U.S. children. N Engl J Med. 2015;372:835–845. , , , et al.;
- American Heart Association. 2005 American Heart Association (AHA) guidelines for cardiopulmonary resuscitation (CPR) and emergency cardiovascular care (ECC) of pediatric and neonatal patients: pediatric basic life support. Pediatrics. 2006;117:e989–e1004.
- Development and initial validation of the Bedside Paediatric Early Warning System score. Crit Care. 2009;13:R135. , , .
- Centers for Medicare and Medicaid Services. Regulations and guidance. EHR incentive programs. Available at: http://www.cms.gov/Regulations‐and‐Guidance/Legislation/EHRIncentivePrograms/index.html. Accessed February 20, 2015
- Development of heart and respiratory rate percentile curves for hospitalized children. Pediatrics. 2013;131:e1150–e1157. , , , , , .
- Length of stay after reaching clinical stability drives hospital costs associated with adult community‐acquired pneumonia. Scand J Infect Dis. 2013;45:219–226. , , , et al.
- Healthcare Cost and Utilization Project. Available at: http://www.ahrq.gov/research/data/hcup/index.html. Accessed February 1, 2014.
- Time to clinical stability in patients hospitalized with community‐acquired pneumonia: implications for practice guidelines. JAMA. 1998;279:1452–1457. , , , et al.
- Neumofail Group. Reaching stability in community‐acquired pneumonia: the effects of the severity of disease, treatment, and the characteristics of patients. Clin Infect Dis. 2004;39:1783–1790. , , , et al.;
- Community‐Acquired Pneumonia Organization. The pneumonia severity index predicts time to clinical stability in patients with community‐acquired pneumonia. Int J Tuberc Lung Dis. 2006;10:739–743. , , , et al.;
- Efficacy of corticosteroids in community‐acquired pneumonia: a randomized double‐blinded clinical trial. Am J Respir Crit Care Med. 2010;181:975–982. , , , , .
- Early administration of antibiotics does not shorten time to clinical stability in patients with moderate‐to‐severe community‐acquired pneumonia. Chest 2003;124:1798–1804. , , , et al.
- A comparison between time to clinical stability in community‐acquired aspiration pneumonia and community‐acquired pneumonia. Intern Emerg Med. 2014;9:143–150. , , , .
- Community‐Acquired Pneumonia Organization (CAPO) Investigators. A worldwide perspective of atypical pathogens in community‐acquired pneumonia. Am J Respir Crit Care Med. 2007;175:1086–1093. , , , et al.;
- CDC EPIC Study Team. Community‐acquired pneumonia requiring hospitalization among U.S. children. N Engl J Med. 2015;372:835–845. , , , et al.;
- American Heart Association. 2005 American Heart Association (AHA) guidelines for cardiopulmonary resuscitation (CPR) and emergency cardiovascular care (ECC) of pediatric and neonatal patients: pediatric basic life support. Pediatrics. 2006;117:e989–e1004.
- Development and initial validation of the Bedside Paediatric Early Warning System score. Crit Care. 2009;13:R135. , , .
- Centers for Medicare and Medicaid Services. Regulations and guidance. EHR incentive programs. Available at: http://www.cms.gov/Regulations‐and‐Guidance/Legislation/EHRIncentivePrograms/index.html. Accessed February 20, 2015
- Development of heart and respiratory rate percentile curves for hospitalized children. Pediatrics. 2013;131:e1150–e1157. , , , , , .
- Length of stay after reaching clinical stability drives hospital costs associated with adult community‐acquired pneumonia. Scand J Infect Dis. 2013;45:219–226. , , , et al.