User login
Obstructive sleep apnea: A better Dx model for primary care
ABSTRACT
Purpose To derive a predictive model for obstructive sleep apnea (OSA) in primary care practice, using home-based overnight oximetry results to refine posttest probability (PTP) of disease after initial risk stratification with the Sleep Apnea Clinical Score (SACS).
Methods We performed secondary analyses on data from a SACS validation cohort, to compare the diagnostic accuracy of 3 overnight oximetry measurements (oxygen desaturation index [ODI], mean saturation, and minimum saturation) in predicting OSA. Receiver operator characteristics (ROC) were computed for each measurement independently and sequentially after risk stratifying with SACS. We examined the implications of oximetry results for OSA PTP for participants categorized as intermediate risk (SACS 6-14; 66/191 participants [35%]; OSA probability 41%). We calculated positive likelihood ratios (LR) for multiple ODI results and determined which ones allowed recalibration to high- or low-risk PTP.
Results Among the 3 oximetry findings, ODI best predicted OSA (area under the curve [AUC], 0.88; 95% confidence interval [CI], 0.83-0.93). An ODI ≥8.4 (likelihood ratio [LR], 4.19; 95% CI, 2.87-6.10) created a PTP of 77%, while an ODI of 0 to <8.4 (LR, 0.19, 95% CI, 0.12-0.33) created a 14% PTP. Sequential application of SACS and ODI results yielded an AUC result of 0.90 (95% CI, 0.85-0.95).
Conclusions SACS risk stratification provides an advantage over clinical gestalt. In those at intermediate risk, ODI results provide a simple and clinically useful way to further refine diagnostic prediction. Sequential use of SACS and selectively employed overnight oximetry may limit unnecessary polysomnography. Oximetry testing should be avoided in patients deemed low or high risk by SACS, as positive results do not substantially recalibrate risk.
Obstructive sleep apnea (OSA) is a prevalent and underdiagnosed condition. The National Sleep Foundation estimates that 18 million Americans have OSA.1 Primary care practice may be the best setting in which to identify OSA, as many of our patients have conditions frequently associated with apnea (eg, hypertension, obesity, diabetes, arrhythmia, and neurologic illness). Up to a third of patients in primary care practice may be at increased risk.2,3
Clinical guidelines of the American Academy of Sleep Medicine (AASM) recommend obtaining a sleep history to evaluate for possible OSA in 3 instances: as part of a routine health maintenance examination, during evaluation of specific complaints associated with OSA (eg, snoring, apnea, daytime sleepiness), and during comprehensive evaluations for individuals with high-risk conditions (ie, obesity, congestive heart failure, refractory hypertension, diabetes, stroke history).4
The American College of Physicians (ACP) Clinical Practice Guideline suggests assessing individuals who have unexplained daytime sleepiness.5 The ACP considers this assessment “High-Value Care,” as “evidence shows that before diagnosis, patients with OSA have higher rates of health care use, more frequent and longer hospital stays, and higher health care costs than after diagnosis.”5
Continue to: We recently validated the diagnostic accuracy...
We recently validated the diagnostic accuracy of the Sleep Apnea Clinical Score (SACS) for use in a primary care patient population suspected of having OSA.6 SACS uses historical and clinical data to derive a score that identifies a patient’s risk level.7 However, as an alternative to the 2 levels described in Flemons’ SACS,7 we propose creating 3 risk strata (FIGURE 17,8). We believe that patients at high risk (SACS ≥15) should be encouraged to undergo sleep evaluations as their posttest probability (PTP) of OSA is 75% to 80%. Individuals at low risk (SACS ≤5; PTP <20%) could receive lifestyle advice and simple clinical interventions that decrease symptoms (eg, weight loss, increased physical activity, sleeping on one’s side). For low-risk patients, clinical observation and reevaluation could take place over time with their primary care provider, without additional testing or referral to specialists.
What about patients at intermediate risk? Many patients suspected of having OSA will be assigned to intermediate risk (SACS 6-14), and their PTP of OSA remains at 40% to 45%, the pre-test level most commonly encountered in suspected OSA. As polysomnography is a limited and expensive clinical resource, intermediate-risk patients would benefit from recalibration of their SACS-based risk assessment using an additional surrogate test such as home-based overnight oximetry. Our internal OSA practice guidelines recommend referral for sleep medicine consultation when oximetry results are abnormal—specifically, an oxygen desaturation index (ODI) of ≥5, a mean saturation less than 89%, and a minimum saturation of 75% or less.
Our objectives in this study were to compare the diagnostic implications of these 3 measurements from home-based overnight oximetry reports and use the most relevant result to derive a predictive model further refining PTP of OSA in a primary care patient population first stratified to intermediate risk by SACS.
METHODS
Subjects
We performed secondary analyses on data obtained from our SACS validation cohort.6 In brief, these were patients suspected of having OSA based on the presence of signs, symptoms, or associated risk factors. One hundred ninety-one patients completed all assessments. Sixty-six of 191 patients (35%) were categorized as intermediate risk (SACS 6-14; OSA probability 41% [27/66]).
Data collection and analyses
Participants completed home-based overnight oximetry using Nonin Model 2500 oximeters (Nonin Medical Inc., Plymouth, Minn). We transferred oximetry results from the sleep lab database to a statistical program for analyses of ODI, mean saturation, and minimal saturation. ODI was defined as the number of 4% drops in saturation from baseline divided by the number of hours of recording time. Although the AASM states that a diagnosis of OSA is confirmed if the number of obstructive events is more than 15 per hour or more than 5 per hour in a patient who reports related symptoms,4 we defined OSA as an apnea-hypopnea index (AHI) of >10 based on polysomnography (as this was the threshold used in the derivation cohort for SACS).7 We demonstrated the predictive ability of SACS at various AHI definitions of OSA in our validation cohort.6 The use of SACS in our validation cohort showed a statistically similar ability to predict OSA at both an AHI of 10 and 20, compared with the derivation cohort.
Continue to: We entered additional information...
We entered additional information reported directly by patients and obtained from their sleep studies into a REDCap database and transferred that to our statistical program. We used descriptive statistics to determine ranges and central tendencies of oximetry results. Receiver operator characteristic (ROC) analyses described the predictive abilities for each oximetry result individually and in serial application with prior SACS determinations. For comparison, we used the area under the ROC curve (AUC) from logistic regression to model the probability of OSA.
We calculated positive likelihood ratios (LR) and 95% confidence intervals (CI) to determine the degree of oximetry abnormality that would recalibrate risk either to a high PTP of OSA (>75%) or a low PTP (<25%). We sorted intermediate-risk SACS scores into quintiles based on ODI results to compare the resulting PTPs of OSA. We applied the PTP of OSA from our previous work (using the SACS score to compute the LR) as the new PTP, estimated the LR based on ODI, and computed an updated PTP of OSA. We also used ROC analysis to determine the optimal cutoff value of the ODI.
Finally, in accordance with our internal clinical practice recommendations, we examined the predictive ability of a “positive” ODI result of ≥5 to recalibrate risk prediction for OSA for patients in the low-risk group. We performed analyses using SAS 9.4 (SAS Institute, Cary, NC).
RESULTS
One hundred ninety-one subjects completed assessments. The median and quartile results for ODI, mean saturation, and minimum saturation are found in TABLE 1. TABLE 2 shows the distribution of patients with positive oximetry results. An ODI of 5 or greater was the most frequent abnormal result (135/191; 70.7%).
We used the AUC to measure the comparative abilities of SACS and the 3 overnight oximetry results in predicting OSA (TABLE 3). ODI results demonstrated the best ability to predict OSA, compared with polysomnography as the relative gold standard (AUC, 0.88; 95% confidence interval [CI], 0.83-0.93). Serial application of SACS and ODI yielded even better diagnostic results (AUC, 0.90; 95% CI, 0.85-0.95).
Continue to: As ODI was found to be the strongest predictor of OSA...
As ODI was found to be the strongest predictor of OSA, we grouped these results in quintiles and calculated positive LRs. TABLE 4 shows their effect on PTP of disease among patients with intermediate risk. An ODI result >10 effected an upward recalibration of disease probability (LR, 2.33; 95% CI, 1.27-4.26). The optimal cutoff of ODI to discriminate between those with and without OSA was determined by ROC analysis. An ODI greater than 8.4 created a PTP of disease of approximately 73% to 77%.
Our internal clinical guidelines recommend referring patients with an ODI of 5 or greater for sleep medicine consultation. We examined the ability of this ODI result to recalibrate disease suspicion for a patient at low risk (SACS ≤5). The LR for ODI of 5 or greater is 2.1, but this only results in a recalibration of risk from 24% pretest probability in our validation cohort to 41% PTP (95% CI, 33-49). This low cutoff for a positive test creates false-positive results more than 40% of the time due to low specificity (0.58). This is insufficient to change the suspicion of disease, resulting only in a shift to intermediate OSA risk.
DISCUSSION
Among 3 different oximetry measurements, an ODI ≥10 best predicts OSA, both independently and when used sequentially after the SACS. ODI was by far the most frequent abnormality on oximetry in our cohort, thereby increasing its utility in clinical decision making. For those subjects at intermediate risk, a cutoff of 10 for the ODI result may be a simple and clinically effective way to recalibrate risk and aid in making referral decisions. (This may also be simpler and more easily remembered by clinicians than the 8.4 ODI results from the ROC analyses.)
Assessment is inadequate without a clinical prediction rule. Unfortunately, providers cannot simply rely on clinical gestalt in diagnosing OSA. In their derivation cohort, Flemens et al examined the LRs created by SACS and by clinician prediction based on history and physical exam.7 The SACS LRs ranged from 5.17 to 0.25, a 20-fold range. This reflected superior diagnostic information compared with subjective physician impression, where LRs ranged from 3.7 to 0.52, a seven-fold range. Myers et al prepared a meta-analysis of 4 different trials that examined physicians’ ability to predict OSA.9 Despite the researchers’ use of experienced sleep medicine doctors, the overall diagnostic accuracy of clinical impression was modest (summary positive LR, 1.7; 95% CI, 1.5-2; I2 = 0%; summary negative LR, 0.67; 95% CI, 0.60-0.74; I2 = 10%; sensitivity, 58%; specificity, 67%). This is similar to reliance on a single clinical sign or symptom to predict OSA.
Wise use of oximetry augments SACS calculation. To limit unnecessary oximetry testing in low- and high-risk groups and to avoid polysomnography in cases of a low PTP of disease, we advocate limiting oximetry testing to individuals in the SACS intermediate-risk group (FIGURE 2) wherein ODI results can potentially recalibrate risk assessment up or down. (Those in the high- risk group should be referred to a sleep medicine specialist.) Our institutional recommendation of using an ODI result of ≥5 as a threshold to increase suspicion of disease requires a caveat for the low-risk group. “Positive” results at that low diagnostic threshold are frequently false.
Continue to: Multiple benefits of SACS
Multiple benefits of SACS. We believe using the SACS calculation during clinical encounters with patients potentially at risk for OSA would increase diagnostic accuracy. Performing risk stratification with SACS should not be an undue burden on providers, and the increased time spent with patients has its own benefits, including helping them better understand their risk. Using this standardized process—augmented, as needed, with overnight ODI assessment—might also encourage more patients to follow through on subsequent recommendations, as their risk is further quantified objectively. Lastly, unnecessary testing with polysomnography could be avoided.
Limitations of our study. This study’s findings were derived from a patient population in a single institution. Replication of the findings from other settings would be helpful.
Looking forward. It is yet unclear if clinicians will embrace these strategies in real-world primary care practice. We have designed an implementation-and-dissemination trial to assess whether family physicians will use the SACS clinical predication rule in everyday practice and whether our evidence-based recommendations about overnight oximetry will be followed. Underlying our suggested clinical evaluation pathway (FIGURE 2) is the belief that there is value gained from sharing the decision-making process with patients. Although we provide new evidence that informs these conversations, the patient’s values and preferences are important when determining the best direction to proceed in the evaluation for suspected OSA. These recommendations are intended to aid, not replace, good clinical judgment.
Home-based sleep testing has become more widely available, is convenient for patients, and is less expensive than lab-based polysomnography. Our study did not directly address the appropriate circumstances for home studies in clinical evaluation. We rely on the expertise of our sleep medicine colleagues to determine which patients are appropriate candidates for home-based studies.
The AASM states that “portable monitors (PM) for the diagnosis of OSA should be [used] only in conjunction with a comprehensive sleep evaluation. Clinical sleep evaluations using PM must be supervised by a practitioner with board certification in sleep medicine or an individual who fulfills the eligibility criteria for the sleep medicine certification examination.”4 Additionally, the group recommends that PM “may be used in the unattended setting as an alternative to polysomnography for the diagnosis of OSA in patients with a high pretest probability of moderate to severe OSA and no comorbid sleep disorder or major comorbid medical disorders.”4
Continue to: GRANT SUPPORT
GRANT SUPPORT
The use of the REDCap database is supported by grant UL1 TR000135. This work was supported by a Mayo Foundation CR-20 grant awarded to Dr. Mookadam as Principal investigator and Dr. Grover as Coinvestigator.
Statistical analyses were supported, in part, by the Department of Family Medicine, Mayo Clinic, Scottsdale, Ariz.
CORRESPONDENCE
Michael Grover, DO, Mayo Clinic Thunderbird Primary Care Center-Family Medicine, 13737 N 92nd Street, Scottsdale, AZ 85260; [email protected]
1. National Sleep Foundation. Sleep apnea. https://sleepfoundation.org/sleep-disorders-problems/sleep-apnea. Accessed September 14, 2018.
2. Grover M, Mookadam M, Armas D, et al. Identifying patients at risk for obstructive sleep apnea in a primary care practice. J Am Board Fam Med. 2011;24:152-160.
3. Mold JW, Quattlebaum C, Schinnerer E, et al. Identification by primary care clinicians of patients with obstructive sleep apnea: a practice-based research network (PBRN) study. J Am Board Fam Med. 2011;24:138-145.
4. Epstein LJ, Kristo D, Strollo PJ, Jr., et al; Adult Obstructive Sleep Apnea Task Force of the American Academy of Sleep Medicine. Clinical guideline for the evaluation, management and long-term care of obstructive sleep apnea in adults. J Clin Sleep Med. 2009;5:263-276.
5. Qaseem A, Dallas P, Owens DK, et al. Diagnosis of obstructive sleep apnea in adults: a clinical practice guideline from the American College of Physicians. Ann Intern Med. 2014;161:210-220.
6. Grover M, Mookadam M, Chang Y-H, et al. Validating the Sleep Apnea Clinical Score for use in primary care populations. Mayo Clin Proc. 2016;91:469-476.
7. Flemons WW, Whitelaw WA, Brant R, et al. Likelihood ratios for a sleep apnea clinical prediction rule. Am J Respir Crit Care Med. 1994;150:1279-1285.
8. Gali B, Whalen FX, Gay PC, et al. Management plan to reduce risks in perioperative care of patients with presumed obstructive sleep apnea syndrome. J Clin Sleep Med. 2007;3:582-588.
9. Myers KA, Mrkobrada M, Simel DL. Does this patient have obstructive sleep apnea?: The rational clinical examination systematic review. JAMA. 2013;310(7):731-741.
ABSTRACT
Purpose To derive a predictive model for obstructive sleep apnea (OSA) in primary care practice, using home-based overnight oximetry results to refine posttest probability (PTP) of disease after initial risk stratification with the Sleep Apnea Clinical Score (SACS).
Methods We performed secondary analyses on data from a SACS validation cohort, to compare the diagnostic accuracy of 3 overnight oximetry measurements (oxygen desaturation index [ODI], mean saturation, and minimum saturation) in predicting OSA. Receiver operator characteristics (ROC) were computed for each measurement independently and sequentially after risk stratifying with SACS. We examined the implications of oximetry results for OSA PTP for participants categorized as intermediate risk (SACS 6-14; 66/191 participants [35%]; OSA probability 41%). We calculated positive likelihood ratios (LR) for multiple ODI results and determined which ones allowed recalibration to high- or low-risk PTP.
Results Among the 3 oximetry findings, ODI best predicted OSA (area under the curve [AUC], 0.88; 95% confidence interval [CI], 0.83-0.93). An ODI ≥8.4 (likelihood ratio [LR], 4.19; 95% CI, 2.87-6.10) created a PTP of 77%, while an ODI of 0 to <8.4 (LR, 0.19, 95% CI, 0.12-0.33) created a 14% PTP. Sequential application of SACS and ODI results yielded an AUC result of 0.90 (95% CI, 0.85-0.95).
Conclusions SACS risk stratification provides an advantage over clinical gestalt. In those at intermediate risk, ODI results provide a simple and clinically useful way to further refine diagnostic prediction. Sequential use of SACS and selectively employed overnight oximetry may limit unnecessary polysomnography. Oximetry testing should be avoided in patients deemed low or high risk by SACS, as positive results do not substantially recalibrate risk.
Obstructive sleep apnea (OSA) is a prevalent and underdiagnosed condition. The National Sleep Foundation estimates that 18 million Americans have OSA.1 Primary care practice may be the best setting in which to identify OSA, as many of our patients have conditions frequently associated with apnea (eg, hypertension, obesity, diabetes, arrhythmia, and neurologic illness). Up to a third of patients in primary care practice may be at increased risk.2,3
Clinical guidelines of the American Academy of Sleep Medicine (AASM) recommend obtaining a sleep history to evaluate for possible OSA in 3 instances: as part of a routine health maintenance examination, during evaluation of specific complaints associated with OSA (eg, snoring, apnea, daytime sleepiness), and during comprehensive evaluations for individuals with high-risk conditions (ie, obesity, congestive heart failure, refractory hypertension, diabetes, stroke history).4
The American College of Physicians (ACP) Clinical Practice Guideline suggests assessing individuals who have unexplained daytime sleepiness.5 The ACP considers this assessment “High-Value Care,” as “evidence shows that before diagnosis, patients with OSA have higher rates of health care use, more frequent and longer hospital stays, and higher health care costs than after diagnosis.”5
Continue to: We recently validated the diagnostic accuracy...
We recently validated the diagnostic accuracy of the Sleep Apnea Clinical Score (SACS) for use in a primary care patient population suspected of having OSA.6 SACS uses historical and clinical data to derive a score that identifies a patient’s risk level.7 However, as an alternative to the 2 levels described in Flemons’ SACS,7 we propose creating 3 risk strata (FIGURE 17,8). We believe that patients at high risk (SACS ≥15) should be encouraged to undergo sleep evaluations as their posttest probability (PTP) of OSA is 75% to 80%. Individuals at low risk (SACS ≤5; PTP <20%) could receive lifestyle advice and simple clinical interventions that decrease symptoms (eg, weight loss, increased physical activity, sleeping on one’s side). For low-risk patients, clinical observation and reevaluation could take place over time with their primary care provider, without additional testing or referral to specialists.
What about patients at intermediate risk? Many patients suspected of having OSA will be assigned to intermediate risk (SACS 6-14), and their PTP of OSA remains at 40% to 45%, the pre-test level most commonly encountered in suspected OSA. As polysomnography is a limited and expensive clinical resource, intermediate-risk patients would benefit from recalibration of their SACS-based risk assessment using an additional surrogate test such as home-based overnight oximetry. Our internal OSA practice guidelines recommend referral for sleep medicine consultation when oximetry results are abnormal—specifically, an oxygen desaturation index (ODI) of ≥5, a mean saturation less than 89%, and a minimum saturation of 75% or less.
Our objectives in this study were to compare the diagnostic implications of these 3 measurements from home-based overnight oximetry reports and use the most relevant result to derive a predictive model further refining PTP of OSA in a primary care patient population first stratified to intermediate risk by SACS.
METHODS
Subjects
We performed secondary analyses on data obtained from our SACS validation cohort.6 In brief, these were patients suspected of having OSA based on the presence of signs, symptoms, or associated risk factors. One hundred ninety-one patients completed all assessments. Sixty-six of 191 patients (35%) were categorized as intermediate risk (SACS 6-14; OSA probability 41% [27/66]).
Data collection and analyses
Participants completed home-based overnight oximetry using Nonin Model 2500 oximeters (Nonin Medical Inc., Plymouth, Minn). We transferred oximetry results from the sleep lab database to a statistical program for analyses of ODI, mean saturation, and minimal saturation. ODI was defined as the number of 4% drops in saturation from baseline divided by the number of hours of recording time. Although the AASM states that a diagnosis of OSA is confirmed if the number of obstructive events is more than 15 per hour or more than 5 per hour in a patient who reports related symptoms,4 we defined OSA as an apnea-hypopnea index (AHI) of >10 based on polysomnography (as this was the threshold used in the derivation cohort for SACS).7 We demonstrated the predictive ability of SACS at various AHI definitions of OSA in our validation cohort.6 The use of SACS in our validation cohort showed a statistically similar ability to predict OSA at both an AHI of 10 and 20, compared with the derivation cohort.
Continue to: We entered additional information...
We entered additional information reported directly by patients and obtained from their sleep studies into a REDCap database and transferred that to our statistical program. We used descriptive statistics to determine ranges and central tendencies of oximetry results. Receiver operator characteristic (ROC) analyses described the predictive abilities for each oximetry result individually and in serial application with prior SACS determinations. For comparison, we used the area under the ROC curve (AUC) from logistic regression to model the probability of OSA.
We calculated positive likelihood ratios (LR) and 95% confidence intervals (CI) to determine the degree of oximetry abnormality that would recalibrate risk either to a high PTP of OSA (>75%) or a low PTP (<25%). We sorted intermediate-risk SACS scores into quintiles based on ODI results to compare the resulting PTPs of OSA. We applied the PTP of OSA from our previous work (using the SACS score to compute the LR) as the new PTP, estimated the LR based on ODI, and computed an updated PTP of OSA. We also used ROC analysis to determine the optimal cutoff value of the ODI.
Finally, in accordance with our internal clinical practice recommendations, we examined the predictive ability of a “positive” ODI result of ≥5 to recalibrate risk prediction for OSA for patients in the low-risk group. We performed analyses using SAS 9.4 (SAS Institute, Cary, NC).
RESULTS
One hundred ninety-one subjects completed assessments. The median and quartile results for ODI, mean saturation, and minimum saturation are found in TABLE 1. TABLE 2 shows the distribution of patients with positive oximetry results. An ODI of 5 or greater was the most frequent abnormal result (135/191; 70.7%).
We used the AUC to measure the comparative abilities of SACS and the 3 overnight oximetry results in predicting OSA (TABLE 3). ODI results demonstrated the best ability to predict OSA, compared with polysomnography as the relative gold standard (AUC, 0.88; 95% confidence interval [CI], 0.83-0.93). Serial application of SACS and ODI yielded even better diagnostic results (AUC, 0.90; 95% CI, 0.85-0.95).
Continue to: As ODI was found to be the strongest predictor of OSA...
As ODI was found to be the strongest predictor of OSA, we grouped these results in quintiles and calculated positive LRs. TABLE 4 shows their effect on PTP of disease among patients with intermediate risk. An ODI result >10 effected an upward recalibration of disease probability (LR, 2.33; 95% CI, 1.27-4.26). The optimal cutoff of ODI to discriminate between those with and without OSA was determined by ROC analysis. An ODI greater than 8.4 created a PTP of disease of approximately 73% to 77%.
Our internal clinical guidelines recommend referring patients with an ODI of 5 or greater for sleep medicine consultation. We examined the ability of this ODI result to recalibrate disease suspicion for a patient at low risk (SACS ≤5). The LR for ODI of 5 or greater is 2.1, but this only results in a recalibration of risk from 24% pretest probability in our validation cohort to 41% PTP (95% CI, 33-49). This low cutoff for a positive test creates false-positive results more than 40% of the time due to low specificity (0.58). This is insufficient to change the suspicion of disease, resulting only in a shift to intermediate OSA risk.
DISCUSSION
Among 3 different oximetry measurements, an ODI ≥10 best predicts OSA, both independently and when used sequentially after the SACS. ODI was by far the most frequent abnormality on oximetry in our cohort, thereby increasing its utility in clinical decision making. For those subjects at intermediate risk, a cutoff of 10 for the ODI result may be a simple and clinically effective way to recalibrate risk and aid in making referral decisions. (This may also be simpler and more easily remembered by clinicians than the 8.4 ODI results from the ROC analyses.)
Assessment is inadequate without a clinical prediction rule. Unfortunately, providers cannot simply rely on clinical gestalt in diagnosing OSA. In their derivation cohort, Flemens et al examined the LRs created by SACS and by clinician prediction based on history and physical exam.7 The SACS LRs ranged from 5.17 to 0.25, a 20-fold range. This reflected superior diagnostic information compared with subjective physician impression, where LRs ranged from 3.7 to 0.52, a seven-fold range. Myers et al prepared a meta-analysis of 4 different trials that examined physicians’ ability to predict OSA.9 Despite the researchers’ use of experienced sleep medicine doctors, the overall diagnostic accuracy of clinical impression was modest (summary positive LR, 1.7; 95% CI, 1.5-2; I2 = 0%; summary negative LR, 0.67; 95% CI, 0.60-0.74; I2 = 10%; sensitivity, 58%; specificity, 67%). This is similar to reliance on a single clinical sign or symptom to predict OSA.
Wise use of oximetry augments SACS calculation. To limit unnecessary oximetry testing in low- and high-risk groups and to avoid polysomnography in cases of a low PTP of disease, we advocate limiting oximetry testing to individuals in the SACS intermediate-risk group (FIGURE 2) wherein ODI results can potentially recalibrate risk assessment up or down. (Those in the high- risk group should be referred to a sleep medicine specialist.) Our institutional recommendation of using an ODI result of ≥5 as a threshold to increase suspicion of disease requires a caveat for the low-risk group. “Positive” results at that low diagnostic threshold are frequently false.
Continue to: Multiple benefits of SACS
Multiple benefits of SACS. We believe using the SACS calculation during clinical encounters with patients potentially at risk for OSA would increase diagnostic accuracy. Performing risk stratification with SACS should not be an undue burden on providers, and the increased time spent with patients has its own benefits, including helping them better understand their risk. Using this standardized process—augmented, as needed, with overnight ODI assessment—might also encourage more patients to follow through on subsequent recommendations, as their risk is further quantified objectively. Lastly, unnecessary testing with polysomnography could be avoided.
Limitations of our study. This study’s findings were derived from a patient population in a single institution. Replication of the findings from other settings would be helpful.
Looking forward. It is yet unclear if clinicians will embrace these strategies in real-world primary care practice. We have designed an implementation-and-dissemination trial to assess whether family physicians will use the SACS clinical predication rule in everyday practice and whether our evidence-based recommendations about overnight oximetry will be followed. Underlying our suggested clinical evaluation pathway (FIGURE 2) is the belief that there is value gained from sharing the decision-making process with patients. Although we provide new evidence that informs these conversations, the patient’s values and preferences are important when determining the best direction to proceed in the evaluation for suspected OSA. These recommendations are intended to aid, not replace, good clinical judgment.
Home-based sleep testing has become more widely available, is convenient for patients, and is less expensive than lab-based polysomnography. Our study did not directly address the appropriate circumstances for home studies in clinical evaluation. We rely on the expertise of our sleep medicine colleagues to determine which patients are appropriate candidates for home-based studies.
The AASM states that “portable monitors (PM) for the diagnosis of OSA should be [used] only in conjunction with a comprehensive sleep evaluation. Clinical sleep evaluations using PM must be supervised by a practitioner with board certification in sleep medicine or an individual who fulfills the eligibility criteria for the sleep medicine certification examination.”4 Additionally, the group recommends that PM “may be used in the unattended setting as an alternative to polysomnography for the diagnosis of OSA in patients with a high pretest probability of moderate to severe OSA and no comorbid sleep disorder or major comorbid medical disorders.”4
Continue to: GRANT SUPPORT
GRANT SUPPORT
The use of the REDCap database is supported by grant UL1 TR000135. This work was supported by a Mayo Foundation CR-20 grant awarded to Dr. Mookadam as Principal investigator and Dr. Grover as Coinvestigator.
Statistical analyses were supported, in part, by the Department of Family Medicine, Mayo Clinic, Scottsdale, Ariz.
CORRESPONDENCE
Michael Grover, DO, Mayo Clinic Thunderbird Primary Care Center-Family Medicine, 13737 N 92nd Street, Scottsdale, AZ 85260; [email protected]
ABSTRACT
Purpose To derive a predictive model for obstructive sleep apnea (OSA) in primary care practice, using home-based overnight oximetry results to refine posttest probability (PTP) of disease after initial risk stratification with the Sleep Apnea Clinical Score (SACS).
Methods We performed secondary analyses on data from a SACS validation cohort, to compare the diagnostic accuracy of 3 overnight oximetry measurements (oxygen desaturation index [ODI], mean saturation, and minimum saturation) in predicting OSA. Receiver operator characteristics (ROC) were computed for each measurement independently and sequentially after risk stratifying with SACS. We examined the implications of oximetry results for OSA PTP for participants categorized as intermediate risk (SACS 6-14; 66/191 participants [35%]; OSA probability 41%). We calculated positive likelihood ratios (LR) for multiple ODI results and determined which ones allowed recalibration to high- or low-risk PTP.
Results Among the 3 oximetry findings, ODI best predicted OSA (area under the curve [AUC], 0.88; 95% confidence interval [CI], 0.83-0.93). An ODI ≥8.4 (likelihood ratio [LR], 4.19; 95% CI, 2.87-6.10) created a PTP of 77%, while an ODI of 0 to <8.4 (LR, 0.19, 95% CI, 0.12-0.33) created a 14% PTP. Sequential application of SACS and ODI results yielded an AUC result of 0.90 (95% CI, 0.85-0.95).
Conclusions SACS risk stratification provides an advantage over clinical gestalt. In those at intermediate risk, ODI results provide a simple and clinically useful way to further refine diagnostic prediction. Sequential use of SACS and selectively employed overnight oximetry may limit unnecessary polysomnography. Oximetry testing should be avoided in patients deemed low or high risk by SACS, as positive results do not substantially recalibrate risk.
Obstructive sleep apnea (OSA) is a prevalent and underdiagnosed condition. The National Sleep Foundation estimates that 18 million Americans have OSA.1 Primary care practice may be the best setting in which to identify OSA, as many of our patients have conditions frequently associated with apnea (eg, hypertension, obesity, diabetes, arrhythmia, and neurologic illness). Up to a third of patients in primary care practice may be at increased risk.2,3
Clinical guidelines of the American Academy of Sleep Medicine (AASM) recommend obtaining a sleep history to evaluate for possible OSA in 3 instances: as part of a routine health maintenance examination, during evaluation of specific complaints associated with OSA (eg, snoring, apnea, daytime sleepiness), and during comprehensive evaluations for individuals with high-risk conditions (ie, obesity, congestive heart failure, refractory hypertension, diabetes, stroke history).4
The American College of Physicians (ACP) Clinical Practice Guideline suggests assessing individuals who have unexplained daytime sleepiness.5 The ACP considers this assessment “High-Value Care,” as “evidence shows that before diagnosis, patients with OSA have higher rates of health care use, more frequent and longer hospital stays, and higher health care costs than after diagnosis.”5
Continue to: We recently validated the diagnostic accuracy...
We recently validated the diagnostic accuracy of the Sleep Apnea Clinical Score (SACS) for use in a primary care patient population suspected of having OSA.6 SACS uses historical and clinical data to derive a score that identifies a patient’s risk level.7 However, as an alternative to the 2 levels described in Flemons’ SACS,7 we propose creating 3 risk strata (FIGURE 17,8). We believe that patients at high risk (SACS ≥15) should be encouraged to undergo sleep evaluations as their posttest probability (PTP) of OSA is 75% to 80%. Individuals at low risk (SACS ≤5; PTP <20%) could receive lifestyle advice and simple clinical interventions that decrease symptoms (eg, weight loss, increased physical activity, sleeping on one’s side). For low-risk patients, clinical observation and reevaluation could take place over time with their primary care provider, without additional testing or referral to specialists.
What about patients at intermediate risk? Many patients suspected of having OSA will be assigned to intermediate risk (SACS 6-14), and their PTP of OSA remains at 40% to 45%, the pre-test level most commonly encountered in suspected OSA. As polysomnography is a limited and expensive clinical resource, intermediate-risk patients would benefit from recalibration of their SACS-based risk assessment using an additional surrogate test such as home-based overnight oximetry. Our internal OSA practice guidelines recommend referral for sleep medicine consultation when oximetry results are abnormal—specifically, an oxygen desaturation index (ODI) of ≥5, a mean saturation less than 89%, and a minimum saturation of 75% or less.
Our objectives in this study were to compare the diagnostic implications of these 3 measurements from home-based overnight oximetry reports and use the most relevant result to derive a predictive model further refining PTP of OSA in a primary care patient population first stratified to intermediate risk by SACS.
METHODS
Subjects
We performed secondary analyses on data obtained from our SACS validation cohort.6 In brief, these were patients suspected of having OSA based on the presence of signs, symptoms, or associated risk factors. One hundred ninety-one patients completed all assessments. Sixty-six of 191 patients (35%) were categorized as intermediate risk (SACS 6-14; OSA probability 41% [27/66]).
Data collection and analyses
Participants completed home-based overnight oximetry using Nonin Model 2500 oximeters (Nonin Medical Inc., Plymouth, Minn). We transferred oximetry results from the sleep lab database to a statistical program for analyses of ODI, mean saturation, and minimal saturation. ODI was defined as the number of 4% drops in saturation from baseline divided by the number of hours of recording time. Although the AASM states that a diagnosis of OSA is confirmed if the number of obstructive events is more than 15 per hour or more than 5 per hour in a patient who reports related symptoms,4 we defined OSA as an apnea-hypopnea index (AHI) of >10 based on polysomnography (as this was the threshold used in the derivation cohort for SACS).7 We demonstrated the predictive ability of SACS at various AHI definitions of OSA in our validation cohort.6 The use of SACS in our validation cohort showed a statistically similar ability to predict OSA at both an AHI of 10 and 20, compared with the derivation cohort.
Continue to: We entered additional information...
We entered additional information reported directly by patients and obtained from their sleep studies into a REDCap database and transferred that to our statistical program. We used descriptive statistics to determine ranges and central tendencies of oximetry results. Receiver operator characteristic (ROC) analyses described the predictive abilities for each oximetry result individually and in serial application with prior SACS determinations. For comparison, we used the area under the ROC curve (AUC) from logistic regression to model the probability of OSA.
We calculated positive likelihood ratios (LR) and 95% confidence intervals (CI) to determine the degree of oximetry abnormality that would recalibrate risk either to a high PTP of OSA (>75%) or a low PTP (<25%). We sorted intermediate-risk SACS scores into quintiles based on ODI results to compare the resulting PTPs of OSA. We applied the PTP of OSA from our previous work (using the SACS score to compute the LR) as the new PTP, estimated the LR based on ODI, and computed an updated PTP of OSA. We also used ROC analysis to determine the optimal cutoff value of the ODI.
Finally, in accordance with our internal clinical practice recommendations, we examined the predictive ability of a “positive” ODI result of ≥5 to recalibrate risk prediction for OSA for patients in the low-risk group. We performed analyses using SAS 9.4 (SAS Institute, Cary, NC).
RESULTS
One hundred ninety-one subjects completed assessments. The median and quartile results for ODI, mean saturation, and minimum saturation are found in TABLE 1. TABLE 2 shows the distribution of patients with positive oximetry results. An ODI of 5 or greater was the most frequent abnormal result (135/191; 70.7%).
We used the AUC to measure the comparative abilities of SACS and the 3 overnight oximetry results in predicting OSA (TABLE 3). ODI results demonstrated the best ability to predict OSA, compared with polysomnography as the relative gold standard (AUC, 0.88; 95% confidence interval [CI], 0.83-0.93). Serial application of SACS and ODI yielded even better diagnostic results (AUC, 0.90; 95% CI, 0.85-0.95).
Continue to: As ODI was found to be the strongest predictor of OSA...
As ODI was found to be the strongest predictor of OSA, we grouped these results in quintiles and calculated positive LRs. TABLE 4 shows their effect on PTP of disease among patients with intermediate risk. An ODI result >10 effected an upward recalibration of disease probability (LR, 2.33; 95% CI, 1.27-4.26). The optimal cutoff of ODI to discriminate between those with and without OSA was determined by ROC analysis. An ODI greater than 8.4 created a PTP of disease of approximately 73% to 77%.
Our internal clinical guidelines recommend referring patients with an ODI of 5 or greater for sleep medicine consultation. We examined the ability of this ODI result to recalibrate disease suspicion for a patient at low risk (SACS ≤5). The LR for ODI of 5 or greater is 2.1, but this only results in a recalibration of risk from 24% pretest probability in our validation cohort to 41% PTP (95% CI, 33-49). This low cutoff for a positive test creates false-positive results more than 40% of the time due to low specificity (0.58). This is insufficient to change the suspicion of disease, resulting only in a shift to intermediate OSA risk.
DISCUSSION
Among 3 different oximetry measurements, an ODI ≥10 best predicts OSA, both independently and when used sequentially after the SACS. ODI was by far the most frequent abnormality on oximetry in our cohort, thereby increasing its utility in clinical decision making. For those subjects at intermediate risk, a cutoff of 10 for the ODI result may be a simple and clinically effective way to recalibrate risk and aid in making referral decisions. (This may also be simpler and more easily remembered by clinicians than the 8.4 ODI results from the ROC analyses.)
Assessment is inadequate without a clinical prediction rule. Unfortunately, providers cannot simply rely on clinical gestalt in diagnosing OSA. In their derivation cohort, Flemens et al examined the LRs created by SACS and by clinician prediction based on history and physical exam.7 The SACS LRs ranged from 5.17 to 0.25, a 20-fold range. This reflected superior diagnostic information compared with subjective physician impression, where LRs ranged from 3.7 to 0.52, a seven-fold range. Myers et al prepared a meta-analysis of 4 different trials that examined physicians’ ability to predict OSA.9 Despite the researchers’ use of experienced sleep medicine doctors, the overall diagnostic accuracy of clinical impression was modest (summary positive LR, 1.7; 95% CI, 1.5-2; I2 = 0%; summary negative LR, 0.67; 95% CI, 0.60-0.74; I2 = 10%; sensitivity, 58%; specificity, 67%). This is similar to reliance on a single clinical sign or symptom to predict OSA.
Wise use of oximetry augments SACS calculation. To limit unnecessary oximetry testing in low- and high-risk groups and to avoid polysomnography in cases of a low PTP of disease, we advocate limiting oximetry testing to individuals in the SACS intermediate-risk group (FIGURE 2) wherein ODI results can potentially recalibrate risk assessment up or down. (Those in the high- risk group should be referred to a sleep medicine specialist.) Our institutional recommendation of using an ODI result of ≥5 as a threshold to increase suspicion of disease requires a caveat for the low-risk group. “Positive” results at that low diagnostic threshold are frequently false.
Continue to: Multiple benefits of SACS
Multiple benefits of SACS. We believe using the SACS calculation during clinical encounters with patients potentially at risk for OSA would increase diagnostic accuracy. Performing risk stratification with SACS should not be an undue burden on providers, and the increased time spent with patients has its own benefits, including helping them better understand their risk. Using this standardized process—augmented, as needed, with overnight ODI assessment—might also encourage more patients to follow through on subsequent recommendations, as their risk is further quantified objectively. Lastly, unnecessary testing with polysomnography could be avoided.
Limitations of our study. This study’s findings were derived from a patient population in a single institution. Replication of the findings from other settings would be helpful.
Looking forward. It is yet unclear if clinicians will embrace these strategies in real-world primary care practice. We have designed an implementation-and-dissemination trial to assess whether family physicians will use the SACS clinical predication rule in everyday practice and whether our evidence-based recommendations about overnight oximetry will be followed. Underlying our suggested clinical evaluation pathway (FIGURE 2) is the belief that there is value gained from sharing the decision-making process with patients. Although we provide new evidence that informs these conversations, the patient’s values and preferences are important when determining the best direction to proceed in the evaluation for suspected OSA. These recommendations are intended to aid, not replace, good clinical judgment.
Home-based sleep testing has become more widely available, is convenient for patients, and is less expensive than lab-based polysomnography. Our study did not directly address the appropriate circumstances for home studies in clinical evaluation. We rely on the expertise of our sleep medicine colleagues to determine which patients are appropriate candidates for home-based studies.
The AASM states that “portable monitors (PM) for the diagnosis of OSA should be [used] only in conjunction with a comprehensive sleep evaluation. Clinical sleep evaluations using PM must be supervised by a practitioner with board certification in sleep medicine or an individual who fulfills the eligibility criteria for the sleep medicine certification examination.”4 Additionally, the group recommends that PM “may be used in the unattended setting as an alternative to polysomnography for the diagnosis of OSA in patients with a high pretest probability of moderate to severe OSA and no comorbid sleep disorder or major comorbid medical disorders.”4
Continue to: GRANT SUPPORT
GRANT SUPPORT
The use of the REDCap database is supported by grant UL1 TR000135. This work was supported by a Mayo Foundation CR-20 grant awarded to Dr. Mookadam as Principal investigator and Dr. Grover as Coinvestigator.
Statistical analyses were supported, in part, by the Department of Family Medicine, Mayo Clinic, Scottsdale, Ariz.
CORRESPONDENCE
Michael Grover, DO, Mayo Clinic Thunderbird Primary Care Center-Family Medicine, 13737 N 92nd Street, Scottsdale, AZ 85260; [email protected]
1. National Sleep Foundation. Sleep apnea. https://sleepfoundation.org/sleep-disorders-problems/sleep-apnea. Accessed September 14, 2018.
2. Grover M, Mookadam M, Armas D, et al. Identifying patients at risk for obstructive sleep apnea in a primary care practice. J Am Board Fam Med. 2011;24:152-160.
3. Mold JW, Quattlebaum C, Schinnerer E, et al. Identification by primary care clinicians of patients with obstructive sleep apnea: a practice-based research network (PBRN) study. J Am Board Fam Med. 2011;24:138-145.
4. Epstein LJ, Kristo D, Strollo PJ, Jr., et al; Adult Obstructive Sleep Apnea Task Force of the American Academy of Sleep Medicine. Clinical guideline for the evaluation, management and long-term care of obstructive sleep apnea in adults. J Clin Sleep Med. 2009;5:263-276.
5. Qaseem A, Dallas P, Owens DK, et al. Diagnosis of obstructive sleep apnea in adults: a clinical practice guideline from the American College of Physicians. Ann Intern Med. 2014;161:210-220.
6. Grover M, Mookadam M, Chang Y-H, et al. Validating the Sleep Apnea Clinical Score for use in primary care populations. Mayo Clin Proc. 2016;91:469-476.
7. Flemons WW, Whitelaw WA, Brant R, et al. Likelihood ratios for a sleep apnea clinical prediction rule. Am J Respir Crit Care Med. 1994;150:1279-1285.
8. Gali B, Whalen FX, Gay PC, et al. Management plan to reduce risks in perioperative care of patients with presumed obstructive sleep apnea syndrome. J Clin Sleep Med. 2007;3:582-588.
9. Myers KA, Mrkobrada M, Simel DL. Does this patient have obstructive sleep apnea?: The rational clinical examination systematic review. JAMA. 2013;310(7):731-741.
1. National Sleep Foundation. Sleep apnea. https://sleepfoundation.org/sleep-disorders-problems/sleep-apnea. Accessed September 14, 2018.
2. Grover M, Mookadam M, Armas D, et al. Identifying patients at risk for obstructive sleep apnea in a primary care practice. J Am Board Fam Med. 2011;24:152-160.
3. Mold JW, Quattlebaum C, Schinnerer E, et al. Identification by primary care clinicians of patients with obstructive sleep apnea: a practice-based research network (PBRN) study. J Am Board Fam Med. 2011;24:138-145.
4. Epstein LJ, Kristo D, Strollo PJ, Jr., et al; Adult Obstructive Sleep Apnea Task Force of the American Academy of Sleep Medicine. Clinical guideline for the evaluation, management and long-term care of obstructive sleep apnea in adults. J Clin Sleep Med. 2009;5:263-276.
5. Qaseem A, Dallas P, Owens DK, et al. Diagnosis of obstructive sleep apnea in adults: a clinical practice guideline from the American College of Physicians. Ann Intern Med. 2014;161:210-220.
6. Grover M, Mookadam M, Chang Y-H, et al. Validating the Sleep Apnea Clinical Score for use in primary care populations. Mayo Clin Proc. 2016;91:469-476.
7. Flemons WW, Whitelaw WA, Brant R, et al. Likelihood ratios for a sleep apnea clinical prediction rule. Am J Respir Crit Care Med. 1994;150:1279-1285.
8. Gali B, Whalen FX, Gay PC, et al. Management plan to reduce risks in perioperative care of patients with presumed obstructive sleep apnea syndrome. J Clin Sleep Med. 2007;3:582-588.
9. Myers KA, Mrkobrada M, Simel DL. Does this patient have obstructive sleep apnea?: The rational clinical examination systematic review. JAMA. 2013;310(7):731-741.
Chest Tube Management
A pneumothorax is a collection of air in the space outside the lungs that is trapped within the thorax. This abnormality can occur spontaneously or as the result of trauma. Traumatic pneumothoraces include those resulting from medical interventions such as a transthoracic and transbronchial needle biopsy, central line placement, and positive‐pressure mechanical ventilation. This group is most accurately described as iatrogenic pneumothorax (IP).[1]
IP can be an expected complication of many routine thoracic procedures, but it can also occur accidentally during procedures near the lung or thoracic cavity. Some IPs may be asymptomatic and go undiagnosed, or their diagnosis may be delayed.[2] The majority of iatrogenic pneumothoraces will resolve without complications, and patients will not require medical attention. A small percentage can, however, expand and have the potential to develop into a tension pneumothorax causing severe respiratory distress and mediastinal shift.[3, 4]
The incidence of IP ranges from 0.11% with mechanical ventilation to 2.68% with thoracentesis, according to an analysis of 7.5 million uniform hospital discharge abstracts from 2000.5 A 2010 systematic review of 24 studies that included 6605 patients suggested a 6.0% incidence of pneumothorax following thoracentesis.[3] The highest risk of IP is seen with computed tomography (CT)‐guided lung biopsy, with 1 series of 1098 biopsies showing a 42% incidence; chest tube evacuation was required in 12% of these cases.[4] A Veterans Administration study of patient safety indicators from 2001 to 2004 found that risk‐adjusted rates of IP were increasing over time.[6] It is unclear whether this increase is due to increasing numbers of interventional procedures or to better rates of detection. IP poses a considerable cost to the medical system, with safety studies finding that patients with IP will stay in the hospital approximately 4 days longer and incur an additional $17,000 in charges.[7]
In addition to this financial burden, the lack of consistency in training and guidelines for management of pneumothorax is thought to add to chest tube‐related complications.[8] In 2001, the American College of Chest Physicians (ACCP) published guidelines for the management of spontaneous pneumothorax that do not specifically address IP.[9] In 2010, the British Thoracic Society (BTS) updated their guidelines and included a brief statement on IP that described a higher incidence for it than for spontaneous pneumothorax and noted its relative ease of management.[10] Despite the lack of specific guidelines dedicated to IPs, common clinical practice is to manage iatrogenic defects in a manner similar to that for spontaneous ones. However, studies have shown that the management of pneumothorax remains diverse and that the adherence to these published guidelines is suboptimal.[10, 11] The BTS guidelines favor needle aspiration as the first‐line treatment,[10] whereas the ACCP recommends drainage with catheters over aspiration.[9]
The possibility of this complication, along with the rising rate of invasive interventions being performed, has led to expanded surveillance criteria for IP. Surveillance imaging, clinical observation, or a combination of the 2 may be required, depending on the institution, the risk of the procedure, and the preference of the treating clinician. The algorithms presented here were designed in alignment with both major society guidelines and with the intention of simplifying the treatment regimen for the ease of adoption by hospitalists.
ETIOLOGY AND RISK FACTORS
The etiology and risk factors for IP are multiple, with the most common being interventional‐based procedures. In 535 Veterans Administration patients, the most common precursor procedures were transthoracic needle biopsy (24%), subclavian vein catheterization (22%), thoracentesis (20%), transbronchial biopsy (10%), pleural biopsy (8%), and positive pressure ventilation.[12] IP can also be a rare complication of pacemaker manipulations,[5] and less commonly, bronchoscopy.[13] Patient factors that increase the risk of pneumothorax in the setting of an intervention include age, chronic obstructive lung disease, primary lung cancer, malignant and parapneumonic pleural effusions, empyema, and chronic corticosteroid use.[4] As might be expected, patients with structural lung disease (eg, emphysema with bullae) and poor healing ability (eg, corticosteroid dependent), tend to have IPs more often and to require more complicated interventions for resolution.[14, 15] In some studies, operator experience seems to be inversely related to the rate of IP, and the use of ultrasound is correlated with lower rates of this complication.[1, 3]
PATIENT PRESENTATIONS AND DIAGNOSIS
Clinical signs and symptoms of a significant pneumothorax vary in severity but most often include dyspnea, tachypnea, chest pain, and pleurisy (see Box 1). Post procedure signs or symptoms require further evaluation with imaging, usually a plain chest radiograph. CT can be useful for further evaluation. Small anterior pneumothoraces may be difficult to detect without lateral radiographic imaging or computed tomogram. Ultrasound is being used more frequently at the bedside to make this diagnosis, and various studies of trauma patients have found that it has good sensitivity and specificity.[16, 17] These results have been validated by a recent meta‐analysis comparing ultrasound to chest radiographs for the detection of pneumothorax among trauma, critically ill, and postprocedural patients.[18] This study demonstrated superior sensitivity and similar specificity for ultrasound versus chest radiographs for detection of pneumothorax. More ominous signs, such as tachycardia or hypotension, can be indicative of tension pneumothorax, which requires emergent evacuation.
MANAGEMENT
Once the diagnosis of pneumothorax has been established, treatment options should be guided by defect size and clinical assessment following a defined treatment algorithm (Figure 1). As emphasized by the BTS and ACCP guidelines, we advocate considering the use of symptoms along with defect size to determine the best management course.
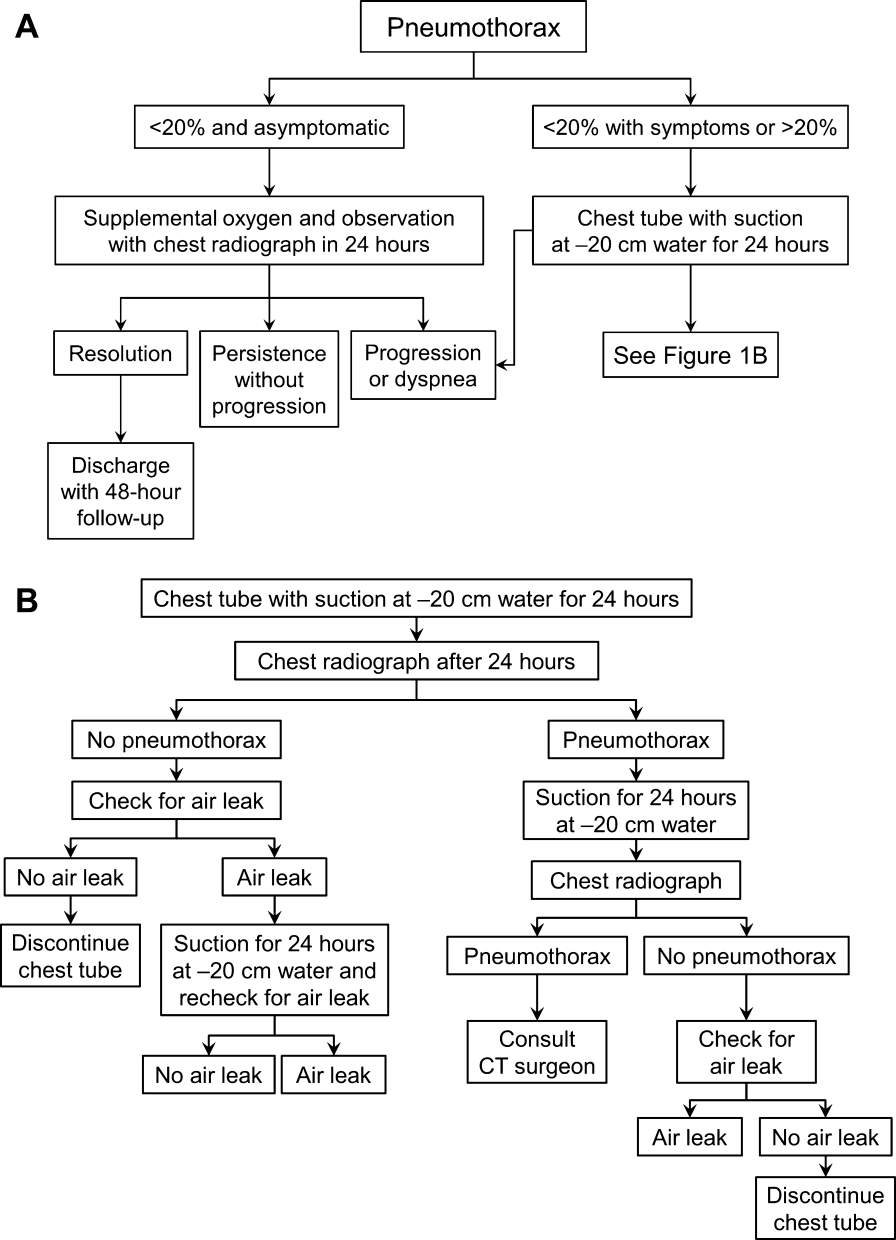
Observation
Defects that involve<20% of the hemithorax in a patient who is clinically asymptomatic and hemodynamically stable can be safely managed by oxygen supplementation and hospital observation. Repeat imaging can be obtained after 12 to 24 hours of defect detection or with symptom change. Patients who display resolution may be discharged home.
Patients who show persistence without progression but are asymptomatic may also be discharged safely, with follow‐up imaging and clinical evaluation 48 hours later.[9, 10] This was demonstrated by Kelly and colleagues,[19] who described the outcomes of 154 patients in a retrospective cohort study. Of the 91 patients treated with outpatient observation, 82 resolved without additional interventions. A recent review article by the same author cites conservative management of small pneumothoraces as being widely accepted.[20] If reimaging shows progression of defect or if the patient becomes more symptomatic, the pneumothorax should be evacuated by 1 of the methods described below.
Aspiration
Aspiration is defined by the ACCP Delphi consensus statement as the removal of pleural air via needle or cannula followed by immediate removal of needle or cannula.[9] This option mandates careful patient selection. It should be considered for small pneumothoraces that cause only mild dyspnea in patients who have no known parenchymal disease. These patients should be observed overnight in the hospital and reimaged 24 hours after aspiration of the pneumothorax. Several authors have reported success with aspiration alone. Yamagami et al.[21] noted the efficacy of manual aspiration immediately after CT‐guided biopsy, with a success rate exceeding 90%. They also noted that evacuated volumes >543 mL correlated with the need for further intervention with a chest tube. This technique is advocated for small pneumothoraces that are recognized shortly after the procedure.
Similarly, Delius and colleagues[22] managed 131 pneumothoraces with aspiration as an alternative to chest tube placement. Of these, 79 were iatrogenic. Aspiration achieved a 75% success rate for all IPs. Small defects defined as <20% of volume had an even higher resolution rate of 87%. Similar findings were demonstrated by Talbot‐Stern et al.[23] in their prospective study of 76 pneumothoraces. Among those that were iatrogenic, 82% resolved after simple aspiration. Faruqi et al.[24] also showed that aspiration is a viable option for IPs. Of the 57 patients with pneumothorax included in their study, 35 were treated with aspiration alone. Iatrogenesis was the culprit in 12 of the 35 manually aspirated cases. Aspiration achieved a success rate of 91.7% in IP. A recent Cochrane database systematic review compared simple aspiration with intercostal tube drainage for primary spontaneous pneumothorax.[25] The authors reported no difference between these methods in terms of success rate, early failure rate, duration of hospital stay, 1‐year success rate, or number of patients who required pleurodesis at 1‐year follow‐up.
Because the algorithms presented in this article were specifically designed for the use by hospitalists, we intentionally omitted aspiration from the decision trees. Most hospitalists would not be expected to evacuate IPs. However, knowledge regarding this option and appropriate follow‐up are valuable to internists, because many interventionalists admit patients to the hospital service for overnight observation. An asymptomatic postaspiration patient, who on subsequent imaging demonstrates resolution or persistence without progression of pneumothorax, may be discharged with 48‐hour follow‐up.
Placement of Catheter or Chest Tube Drainage
Most patients with a clinically significant pneumothorax will require evacuation of the air. Pneumothoraces larger than 20% or that produce symptoms warrant chest tube management and inpatient observation (Figure 1B). Traditionally, large tubes with 20 cm of water on continuous suction are used and have been studied the most widely. Several authors have shown that smaller tubes can effectively drain a pneumothorax.[26, 27, 28, 29] Small‐bore catheters (8F14F), which can be inserted percutaneously, have been shown to provide effective lung re‐expansion with minimal morbidity[8] and may be better tolerated by patients with uncomplicated pneumothoraces (Figure 2). Terzi and colleagues[30] have shown that smaller tubes cause less discomfort to patients at rest, with cough, and at the time of tube removal.
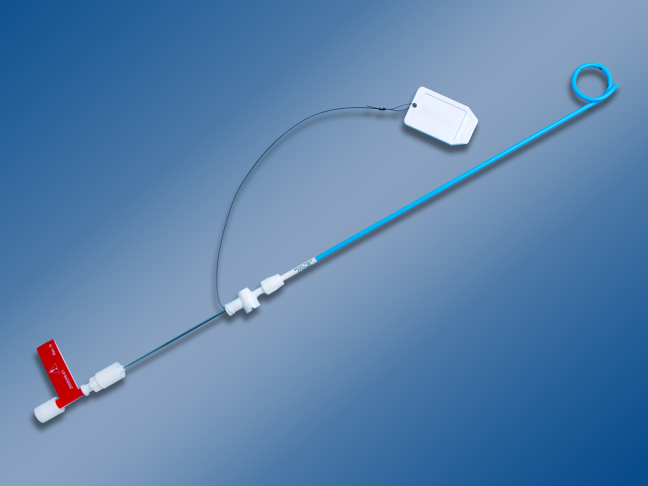
At most US institutions, catheters and chest tubes are connected to all‐purpose drainage systems. Although commercially available through a variety of manufacturers, they share similar design principles because they replicate the 3‐bottle system described in detail elsewhere in the literature.[31] We have limited our discussion to 3 pleural evacuation systems because it is our intention to familiarize hospitalists with the units that they are most likely to encounter. The first 2 systems have been studied and described by Baumann and colleagues[32] as being commonplace and reasonably reliable. These include the Oasis (Atrium Medical Corp., Hudson, NH) (Figure 3A) and the Pleur‐evac (Teleflex Inc., Limerick, PA). The third unit is the Thopaz digital thoracic drainage system (Medela Inc., McHenry, IL) (Figure 3B). The Thopaz is unique in its inclusion of a suction source and digital capability. Although it utilizes the same principles of all pleural evacuation devices, its setup and information output require that one be familiar with its digital format.
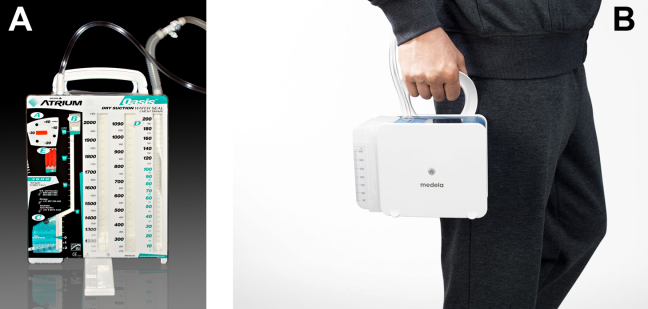
Suction Versus Water Seal
The chest tube should be placed initially to a suction pressure level of 20 cm of water for 24 hours to maximize lung expansion and evacuate all extrapulmonary air. Suction pressure is set on the Pleur‐evac and Atrium drainage systems by a manual dial that reads to a water pressure of 0 to 40 cm. The default setting from the manufacturer is 20 cm of water. This level of suction is present only when the drainage system is connected to a wall or a portable suction device. The only confirmation of suction presence in the Atrium system is the deployment of the orange bellows (located under the dial) to the level of the arrow tip (Figure 3A). The Pleur‐evac system has a red stripe along the circular edge of the dial that appears at the set level of suction when negative pressure is being applied. It is important to be aware that when patients are disconnected from the wall or the portable suction apparatus, they are on water seal or gravity. These terms are synonymous with no suction. On the Thopaz, a digital menu directs operation, and levels of suction can be selected from water seal (no suction) up to 40 cm of water. We recommend using suction to 20 cm of water given the scarce evidence supporting higher levels of negative pressure. Some clinicians prefer placing patients on water seal for some time before moving toward tube discontinuance, but this is a matter of preference, and no substantial evidence exists to show that any 1 method is superior.[8, 33]
Assessing for Air Leak
If there is improvement or resolution of the pneumothorax after 24 hours, the presence of an air leak should be assessed; if no leak is present, the chest tube can be safely removed. In the context of chest tubes, the term air leak refers to residual air between the lung and the chest wall. It is possible to see resolution of a pneumothorax on chest radiographs and still have an air leak. This situation is created by a perfect balance between the pleural air evacuation by the catheter and the flow of air exiting from the lung puncture. This would result in reaccumulation of the pneumothorax if the chest tube is removed prematurely. It should also be kept in mind that chest radiographs may miss a small pneumothorax given their relatively low sensitivity.[18] Therefore, the absence of an air leak needs to be documented before the chest tube is discontinued. Depending on the type of drainage system (Atrium, Pleur‐evac, or Thopaz), this assessment can be done in several ways. All systems can be assessed for air leak by clamping the actual chest tube for 2 to 4 hours and then repeating the chest radiograph. Clamping a chest tube simulates the condition of not having a chest tube. Chest tubes should never be clamped without supervision and only with the knowledge of nursing personnel. The onset of chest pain or dyspnea in a patient with a clamped tube mandates immediate removal of the clamp and a return to suction. A repeat chest radiograph showing reaccumulation or expansion of the pneumothorax after clamping indicates that the air leak has not resolved and the chest tube must remain in place and returned to suction. Simpler and more time‐efficient methods of detecting air leaks are available with both cardiothoracic drainage systems.
For the Atrium and Pleur‐evac models, there is a graded panel through which one can visualize air leaks being funneled through water (Figure 4A). Having the patient cough several times or perform a Valsalva maneuver should release any air trapped within the chest into this chamber, where bubbles can be visualized as they travel through the water. The presence of bubbles indicates the presence of residual air in the chest, pointing to a possible leak. In contrast, the Thopaz system offers a graphical display of the air flowing into the system that can be reviewed over the 24‐hour period. When the graph line reaches a 0 flatline graph, no airflow is being detected and no air leakage is suspected (Figure 4B). If no air leaks are detected, the chest tube may be discontinued. Those patients with a failed air leak test should have their chest tubes continued under suction for another 24 hours, with the above tests then repeated. The same holds true for those patients with persistent pneumothorax at 24 hours.
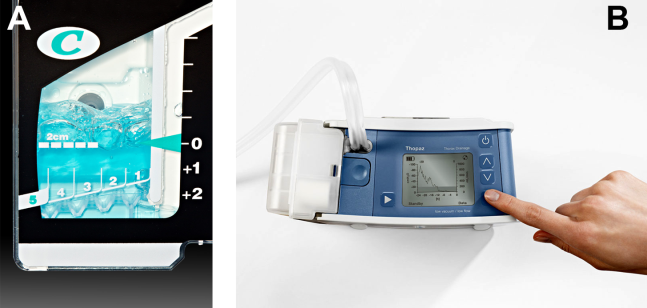
Removal of chest tubes is a simple process that requires the tube to be pulled out of the patient without allowing air to enter the site where the tube was present and where it entered the thorax. Most interventionalists will discontinue the tubes that they have placed. Some small catheters have an internal string that has to be released so the catheter will straighten and pull out easily. Knowledge of the type of catheter or tube that was placed is critical before removal to prevent complications and patient discomfort. Standard chest tubes are straight, smooth plastic and pull out easily but require rapid occlusion of the larger puncture site in the chest wall with an occlusive dressing that often includes petroleum or water‐soluble gel. Some physicians will leave a suture tie when placing the chest tube so that it can be tied down to occlude the site instead of using a dressing.
Consultation of the Cardiothoracic Surgeon or Interventional Pulmonologist
We recommend the involvement of cardiothoracic surgery or interventional pulmonology for patients with nonresolving pneumothorax lasting longer than 48 hours because additional procedures may be necessary. One of the rare but serious complications of a persistent pneumothorax is the formation of a bronchopleural fistula. This communication between the bronchial tree and the pleural space can lead to significant morbidity and mortality. The treatment of a bronchopleural fistula includes medical and surgical options that are beyond the scope of this article but require the expertise of a cardiothoracic surgeon or interventional pulmonologist.[34] Most patients who will not require additional procedures will heal within 48 hours.[11, 35] Decisions regarding more invasive treatment measures can then be made as necessary.[26]
PRACTICAL TIPS
Hospitalists caring for patients with chest tubes are often asked to troubleshoot at the bedside. Scenarios that may be encountered include nonfunctioning tubes, catheter migration, and tube discomfort. Ensuring patency of the tube entails visualizing the tube from the point of entry into the chest wall to the collection chamber and inspecting for kinks or debris clogging the tube. Smaller catheters can be easily kinked during patient positioning and can become clogged. Respiratory variation, which is the movement of the column of fluid in the collection chamber or in the tubing with inspiration and expiration, suggests that the chest tube is patent. This should be part of the daily examination in a patient with a chest tube, and it should also be the first step in assessing sudden dyspnea, hypoxia, pain, or hemodynamic instability. Clogged tubes should be referred to the interventionalists or other physicians who placed them. Chest tubes are typically sutured at the site of entry and securely bandaged to avoid migration but occasionally can be dislodged. This should prompt placement of another tube by an experienced operator. Last, chest tubes can be uncomfortable for patients who may require systemic analgesics. Additionally, tube positioning may ease some of the discomfort. Chest tubes are commonly placed along the midaxillary line and the posterior thorax, leading to discomfort in the recumbent position. Directing the tube anteriorly helps ease some of the discomfort. This can be done using all‐purpose sponges to build a barrier between the skin and the chest tube as it is directed anteriorly. Additional sponges are placed above the tube for extra protection. The gentle curve accomplished by padding the underside of the tube also keeps the tube patent by avoiding sharp kinks as the catheter exits the thorax.
FUTURE TRENDS
Future trends in the management of IP may include shorter duration of tube management (14 hours of suction with air leak evaluation and removal) and the use of even smaller catheters. Outpatient management of IP with small pigtail catheters or small‐caliber tubes in addition to 1‐way valves is also being investigated. The benefits of these practices may include greater patient comfort and lower cost. These approaches will need larger‐scale replication and careful patient selection before they become standard practice.[28, 36]
Ultrasound can be used to assess the presence and size of pneumothoraces that are difficult to visualize by standard chest radiographs. Several studies have established ultrasonography as an effective method of diagnosing pneumothorax and have shown it to have superior sensitivity compared with chest radiography.[1, 18] In the future, the use of ultrasound will likely be more widespread given its performance, portability, ease of use, and relatively low cost.
SUMMARY
IP is a known and costly complication of many medical procedures. The aforementioned algorithms help simplify the management of chest tubes for hospitalists caring for patients with this common complication. This stepwise approach may not only help curtail added expenses related to IPs by decreasing the length of inpatient stays but may also improve patient satisfaction.
Acknowledgments
Disclosure: Mayo does not endorse the products mentioned in this article. The authors report no conflicts of interest.
Box
Clinical Signs and Symptoms of Pneumothorax
Dyspnea
Pleuritic chest pain
Tachypnea
Hypoxia
Decreased breath sounds on affected side
Hyper‐resonant percussion on affected side
Subcutaneous emphysema
- Management of pneumothorax. Semin Respir Crit Care Med. 2010;31(6):769–780. , .
- Pneumothorax. Respirology. 2004;9(2):157–164. , .
- Pneumothorax following thoracentesis: a systematic review and meta‐analysis. Arch Intern Med. 2010;170(4):332–339. , , , .
- Incidence of and risk factors for pneumothorax and chest tube placement after CT fluoroscopy‐guided percutaneous lung biopsy: retrospective analysis of the procedures conducted over a 9‐year period. AJR Am J Roentgenol. 2010;194(3):809–814. , , , et al.
- Accidental iatrogenic pneumothorax in hospitalized patients. Med Care. 2006;44(2):182–186. , , .
- Tracking rates of patient safety indicators over time: lessons from the Veterans Administration. Med Care. 2006;44(9):850–861. , , , et al.
- Excess length of stay, charges, and mortality attributable to medical injuries during hospitalization. JAMA. 2003;290(14):1868–1874. , .
- Preliminary report of a prospective, randomized trial of underwater seal for spontaneous and iatrogenic pneumothorax. J Am Coll Surg. 2007;204(1):84–90. , , , , .
- AACP Pneumothorax Consensus Group. Management of spontaneous pneumothorax: an American College of Chest Physicians Delphi consensus statement. Chest. 2001;119(2):590–602. , , , et al.;
- BTS Pleural Disease Guideline Group. Management of spontaneous pneumothorax: British Thoracic Society Pleural Disease Guideline 2010. Thorax. 2010;65(suppl 2):ii18–ii31. , , ;
- The clinician's perspective on pneumothorax management. Chest. 1997;112(3):822–828. , .
- Iatrogenic pneumothorax: etiology and morbidity: results of a Department of Veterans Affairs Cooperative Study. Respiration. 1992;59(4):215–220. , , , .
- Severe complications of bronchoscopy. Respiration. 2008;76(4):429–433. , , , , , .
- Incidence and risk factors of delayed pneumothorax after transthoracic needle biopsy of the lung. Chest. 2004;126(5):1516–1521. , , , et al.
- Factors associated with pneumothorax and pneumothorax requiring treatment after percutaneous lung biopsy in 443 consecutive patients. J Vasc Interv Radiol. 2004;15(5):479–483. , , , , , .
- Ultrasound detection of pneumothorax compared with chest X‐ray and computed tomography scan. Am Surg. 2011;77(4):480–484. , .
- Sensitivity of bedside ultrasound and supine anteroposterior chest radiographs for the identification of pneumothorax after blunt trauma. Acad Emerg Med. 2010;17(1):11–17. , .
- Diagnosis of pneumothorax by radiography and ultrasonography: a meta‐analysis. Chest. 2011;140(4):859–866. , , , , .
- Outcomes of emergency department patients treated for primary spontaneous pneumothorax. Chest. 2008;134(5):1033–1036. , , .
- Review of management of primary spontaneous pneumothorax: is the best evidence clearer 15 years on? Emerg Med Australas. 2007;19(4):303–308. .
- Efficacy of manual aspiration immediately after complicated pneumothorax in CT‐guided lung biopsy. J Vasc Interv Radiol. 2005;16(4):477–483. , , , , , .
- Catheter aspiration for simple pneumothorax: experience with 114 patients. Arch Surg. 1998;124(7):833–836. , , , , , .
- Catheter aspiration for simple pneumothorax. J Emerg Med. 1986;4(6):437–442. , , , , .
- Role of simple needle aspiration in the management of pneumothorax. Indian J Chest Dis Allied Sci. 2004;46(3):183–190. , , , .
- Simple aspiration versus intercostal tube drainage for primary spontaneous pneumothorax in adults. Cochrane Database Syst Rev. 2007;(1):CD004479. , , .
- Evaluation of conventional chest tube therapy for iatrogenic pneumothorax. Chest. 1993;104(6):1770–1772. , , , .
- Outpatient treatment of iatrogenic pneumothorax after needle biopsy. Radiology. 1997;205(1):249–252. , , , .
- Outpatient management of postbiopsy pneumothorax with small‐caliber chest tubes: factors affecting the need for prolonged drainage and additional interventions. Cardiovasc Intervent Radiol. 2008;31(2):342–348. , , , , , .
- Management of primary and secondary pneumothorax using a small‐bore thoracic catheter. Interact Cardiovasc Thorac Surg. 2010;11(2):146–149. , .
- The use of flexible spiral drains after non‐cardiac thoracic surgery: a clinical study. Eur J Cardiothorac Surg. 2005;27(1):134–137. , , , et al.
- Pleural Diseases. 5th ed. Philadelphia: PA: Lippincott Williams 2007. .
- Comparison of function of commercially available pleural drainage units and catheters. Chest. 2003;123(6):1878–1886. , , , .
- Catheter drainage of spontaneous pneumothorax: suction or no suction, early or late removal? Thorax. 1982;37(1):46–48. , .
- Bronchopleural fistulas: an overview of the problem with special focus on endoscopic management. Chest. 2005;128(6):3955–3965. , .
- Timing of invasive procedures in therapy for primary and secondary spontaneous pneumothorax. Arch Surg. 1991;126(6):764–766. , , , .
- A treatment algorithm for pneumothoraces complicating central venous catheter insertion. Am J Surg. 2000;180(6):523–526. , , , , .
A pneumothorax is a collection of air in the space outside the lungs that is trapped within the thorax. This abnormality can occur spontaneously or as the result of trauma. Traumatic pneumothoraces include those resulting from medical interventions such as a transthoracic and transbronchial needle biopsy, central line placement, and positive‐pressure mechanical ventilation. This group is most accurately described as iatrogenic pneumothorax (IP).[1]
IP can be an expected complication of many routine thoracic procedures, but it can also occur accidentally during procedures near the lung or thoracic cavity. Some IPs may be asymptomatic and go undiagnosed, or their diagnosis may be delayed.[2] The majority of iatrogenic pneumothoraces will resolve without complications, and patients will not require medical attention. A small percentage can, however, expand and have the potential to develop into a tension pneumothorax causing severe respiratory distress and mediastinal shift.[3, 4]
The incidence of IP ranges from 0.11% with mechanical ventilation to 2.68% with thoracentesis, according to an analysis of 7.5 million uniform hospital discharge abstracts from 2000.5 A 2010 systematic review of 24 studies that included 6605 patients suggested a 6.0% incidence of pneumothorax following thoracentesis.[3] The highest risk of IP is seen with computed tomography (CT)‐guided lung biopsy, with 1 series of 1098 biopsies showing a 42% incidence; chest tube evacuation was required in 12% of these cases.[4] A Veterans Administration study of patient safety indicators from 2001 to 2004 found that risk‐adjusted rates of IP were increasing over time.[6] It is unclear whether this increase is due to increasing numbers of interventional procedures or to better rates of detection. IP poses a considerable cost to the medical system, with safety studies finding that patients with IP will stay in the hospital approximately 4 days longer and incur an additional $17,000 in charges.[7]
In addition to this financial burden, the lack of consistency in training and guidelines for management of pneumothorax is thought to add to chest tube‐related complications.[8] In 2001, the American College of Chest Physicians (ACCP) published guidelines for the management of spontaneous pneumothorax that do not specifically address IP.[9] In 2010, the British Thoracic Society (BTS) updated their guidelines and included a brief statement on IP that described a higher incidence for it than for spontaneous pneumothorax and noted its relative ease of management.[10] Despite the lack of specific guidelines dedicated to IPs, common clinical practice is to manage iatrogenic defects in a manner similar to that for spontaneous ones. However, studies have shown that the management of pneumothorax remains diverse and that the adherence to these published guidelines is suboptimal.[10, 11] The BTS guidelines favor needle aspiration as the first‐line treatment,[10] whereas the ACCP recommends drainage with catheters over aspiration.[9]
The possibility of this complication, along with the rising rate of invasive interventions being performed, has led to expanded surveillance criteria for IP. Surveillance imaging, clinical observation, or a combination of the 2 may be required, depending on the institution, the risk of the procedure, and the preference of the treating clinician. The algorithms presented here were designed in alignment with both major society guidelines and with the intention of simplifying the treatment regimen for the ease of adoption by hospitalists.
ETIOLOGY AND RISK FACTORS
The etiology and risk factors for IP are multiple, with the most common being interventional‐based procedures. In 535 Veterans Administration patients, the most common precursor procedures were transthoracic needle biopsy (24%), subclavian vein catheterization (22%), thoracentesis (20%), transbronchial biopsy (10%), pleural biopsy (8%), and positive pressure ventilation.[12] IP can also be a rare complication of pacemaker manipulations,[5] and less commonly, bronchoscopy.[13] Patient factors that increase the risk of pneumothorax in the setting of an intervention include age, chronic obstructive lung disease, primary lung cancer, malignant and parapneumonic pleural effusions, empyema, and chronic corticosteroid use.[4] As might be expected, patients with structural lung disease (eg, emphysema with bullae) and poor healing ability (eg, corticosteroid dependent), tend to have IPs more often and to require more complicated interventions for resolution.[14, 15] In some studies, operator experience seems to be inversely related to the rate of IP, and the use of ultrasound is correlated with lower rates of this complication.[1, 3]
PATIENT PRESENTATIONS AND DIAGNOSIS
Clinical signs and symptoms of a significant pneumothorax vary in severity but most often include dyspnea, tachypnea, chest pain, and pleurisy (see Box 1). Post procedure signs or symptoms require further evaluation with imaging, usually a plain chest radiograph. CT can be useful for further evaluation. Small anterior pneumothoraces may be difficult to detect without lateral radiographic imaging or computed tomogram. Ultrasound is being used more frequently at the bedside to make this diagnosis, and various studies of trauma patients have found that it has good sensitivity and specificity.[16, 17] These results have been validated by a recent meta‐analysis comparing ultrasound to chest radiographs for the detection of pneumothorax among trauma, critically ill, and postprocedural patients.[18] This study demonstrated superior sensitivity and similar specificity for ultrasound versus chest radiographs for detection of pneumothorax. More ominous signs, such as tachycardia or hypotension, can be indicative of tension pneumothorax, which requires emergent evacuation.
MANAGEMENT
Once the diagnosis of pneumothorax has been established, treatment options should be guided by defect size and clinical assessment following a defined treatment algorithm (Figure 1). As emphasized by the BTS and ACCP guidelines, we advocate considering the use of symptoms along with defect size to determine the best management course.
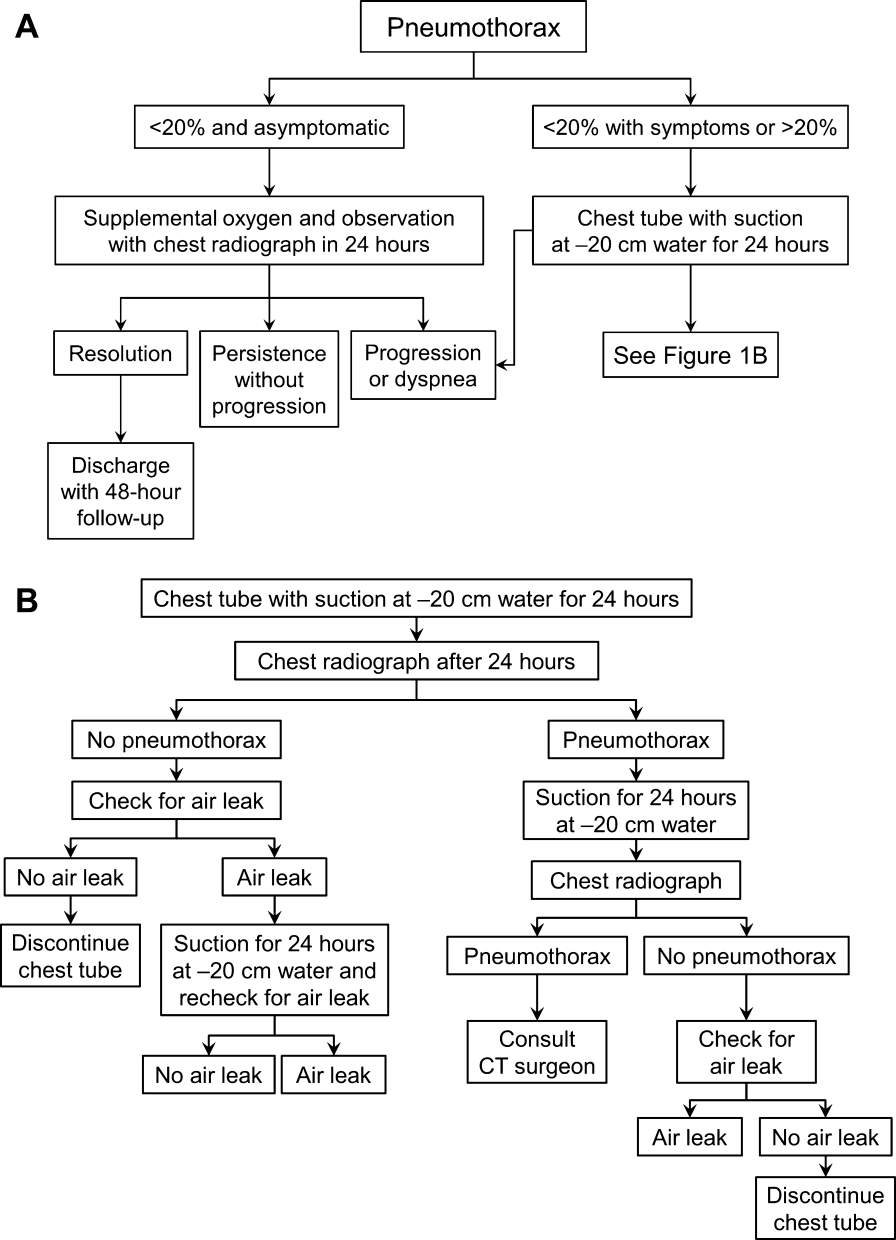
Observation
Defects that involve<20% of the hemithorax in a patient who is clinically asymptomatic and hemodynamically stable can be safely managed by oxygen supplementation and hospital observation. Repeat imaging can be obtained after 12 to 24 hours of defect detection or with symptom change. Patients who display resolution may be discharged home.
Patients who show persistence without progression but are asymptomatic may also be discharged safely, with follow‐up imaging and clinical evaluation 48 hours later.[9, 10] This was demonstrated by Kelly and colleagues,[19] who described the outcomes of 154 patients in a retrospective cohort study. Of the 91 patients treated with outpatient observation, 82 resolved without additional interventions. A recent review article by the same author cites conservative management of small pneumothoraces as being widely accepted.[20] If reimaging shows progression of defect or if the patient becomes more symptomatic, the pneumothorax should be evacuated by 1 of the methods described below.
Aspiration
Aspiration is defined by the ACCP Delphi consensus statement as the removal of pleural air via needle or cannula followed by immediate removal of needle or cannula.[9] This option mandates careful patient selection. It should be considered for small pneumothoraces that cause only mild dyspnea in patients who have no known parenchymal disease. These patients should be observed overnight in the hospital and reimaged 24 hours after aspiration of the pneumothorax. Several authors have reported success with aspiration alone. Yamagami et al.[21] noted the efficacy of manual aspiration immediately after CT‐guided biopsy, with a success rate exceeding 90%. They also noted that evacuated volumes >543 mL correlated with the need for further intervention with a chest tube. This technique is advocated for small pneumothoraces that are recognized shortly after the procedure.
Similarly, Delius and colleagues[22] managed 131 pneumothoraces with aspiration as an alternative to chest tube placement. Of these, 79 were iatrogenic. Aspiration achieved a 75% success rate for all IPs. Small defects defined as <20% of volume had an even higher resolution rate of 87%. Similar findings were demonstrated by Talbot‐Stern et al.[23] in their prospective study of 76 pneumothoraces. Among those that were iatrogenic, 82% resolved after simple aspiration. Faruqi et al.[24] also showed that aspiration is a viable option for IPs. Of the 57 patients with pneumothorax included in their study, 35 were treated with aspiration alone. Iatrogenesis was the culprit in 12 of the 35 manually aspirated cases. Aspiration achieved a success rate of 91.7% in IP. A recent Cochrane database systematic review compared simple aspiration with intercostal tube drainage for primary spontaneous pneumothorax.[25] The authors reported no difference between these methods in terms of success rate, early failure rate, duration of hospital stay, 1‐year success rate, or number of patients who required pleurodesis at 1‐year follow‐up.
Because the algorithms presented in this article were specifically designed for the use by hospitalists, we intentionally omitted aspiration from the decision trees. Most hospitalists would not be expected to evacuate IPs. However, knowledge regarding this option and appropriate follow‐up are valuable to internists, because many interventionalists admit patients to the hospital service for overnight observation. An asymptomatic postaspiration patient, who on subsequent imaging demonstrates resolution or persistence without progression of pneumothorax, may be discharged with 48‐hour follow‐up.
Placement of Catheter or Chest Tube Drainage
Most patients with a clinically significant pneumothorax will require evacuation of the air. Pneumothoraces larger than 20% or that produce symptoms warrant chest tube management and inpatient observation (Figure 1B). Traditionally, large tubes with 20 cm of water on continuous suction are used and have been studied the most widely. Several authors have shown that smaller tubes can effectively drain a pneumothorax.[26, 27, 28, 29] Small‐bore catheters (8F14F), which can be inserted percutaneously, have been shown to provide effective lung re‐expansion with minimal morbidity[8] and may be better tolerated by patients with uncomplicated pneumothoraces (Figure 2). Terzi and colleagues[30] have shown that smaller tubes cause less discomfort to patients at rest, with cough, and at the time of tube removal.
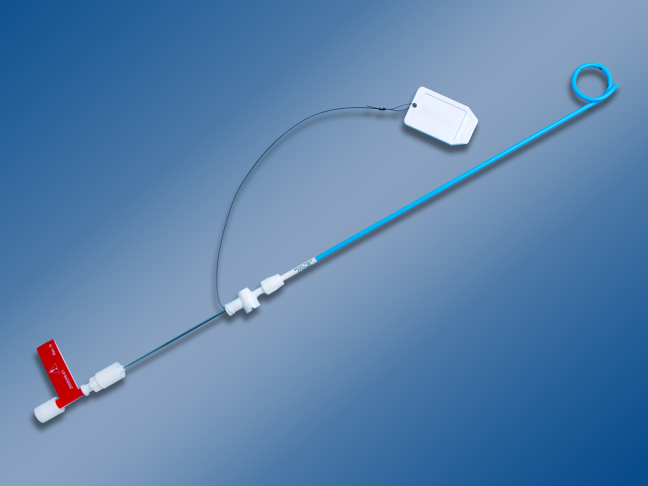
At most US institutions, catheters and chest tubes are connected to all‐purpose drainage systems. Although commercially available through a variety of manufacturers, they share similar design principles because they replicate the 3‐bottle system described in detail elsewhere in the literature.[31] We have limited our discussion to 3 pleural evacuation systems because it is our intention to familiarize hospitalists with the units that they are most likely to encounter. The first 2 systems have been studied and described by Baumann and colleagues[32] as being commonplace and reasonably reliable. These include the Oasis (Atrium Medical Corp., Hudson, NH) (Figure 3A) and the Pleur‐evac (Teleflex Inc., Limerick, PA). The third unit is the Thopaz digital thoracic drainage system (Medela Inc., McHenry, IL) (Figure 3B). The Thopaz is unique in its inclusion of a suction source and digital capability. Although it utilizes the same principles of all pleural evacuation devices, its setup and information output require that one be familiar with its digital format.
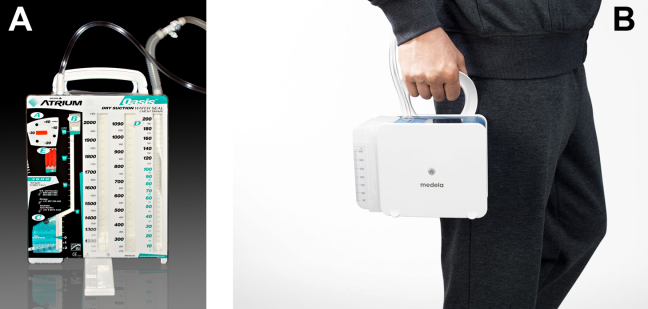
Suction Versus Water Seal
The chest tube should be placed initially to a suction pressure level of 20 cm of water for 24 hours to maximize lung expansion and evacuate all extrapulmonary air. Suction pressure is set on the Pleur‐evac and Atrium drainage systems by a manual dial that reads to a water pressure of 0 to 40 cm. The default setting from the manufacturer is 20 cm of water. This level of suction is present only when the drainage system is connected to a wall or a portable suction device. The only confirmation of suction presence in the Atrium system is the deployment of the orange bellows (located under the dial) to the level of the arrow tip (Figure 3A). The Pleur‐evac system has a red stripe along the circular edge of the dial that appears at the set level of suction when negative pressure is being applied. It is important to be aware that when patients are disconnected from the wall or the portable suction apparatus, they are on water seal or gravity. These terms are synonymous with no suction. On the Thopaz, a digital menu directs operation, and levels of suction can be selected from water seal (no suction) up to 40 cm of water. We recommend using suction to 20 cm of water given the scarce evidence supporting higher levels of negative pressure. Some clinicians prefer placing patients on water seal for some time before moving toward tube discontinuance, but this is a matter of preference, and no substantial evidence exists to show that any 1 method is superior.[8, 33]
Assessing for Air Leak
If there is improvement or resolution of the pneumothorax after 24 hours, the presence of an air leak should be assessed; if no leak is present, the chest tube can be safely removed. In the context of chest tubes, the term air leak refers to residual air between the lung and the chest wall. It is possible to see resolution of a pneumothorax on chest radiographs and still have an air leak. This situation is created by a perfect balance between the pleural air evacuation by the catheter and the flow of air exiting from the lung puncture. This would result in reaccumulation of the pneumothorax if the chest tube is removed prematurely. It should also be kept in mind that chest radiographs may miss a small pneumothorax given their relatively low sensitivity.[18] Therefore, the absence of an air leak needs to be documented before the chest tube is discontinued. Depending on the type of drainage system (Atrium, Pleur‐evac, or Thopaz), this assessment can be done in several ways. All systems can be assessed for air leak by clamping the actual chest tube for 2 to 4 hours and then repeating the chest radiograph. Clamping a chest tube simulates the condition of not having a chest tube. Chest tubes should never be clamped without supervision and only with the knowledge of nursing personnel. The onset of chest pain or dyspnea in a patient with a clamped tube mandates immediate removal of the clamp and a return to suction. A repeat chest radiograph showing reaccumulation or expansion of the pneumothorax after clamping indicates that the air leak has not resolved and the chest tube must remain in place and returned to suction. Simpler and more time‐efficient methods of detecting air leaks are available with both cardiothoracic drainage systems.
For the Atrium and Pleur‐evac models, there is a graded panel through which one can visualize air leaks being funneled through water (Figure 4A). Having the patient cough several times or perform a Valsalva maneuver should release any air trapped within the chest into this chamber, where bubbles can be visualized as they travel through the water. The presence of bubbles indicates the presence of residual air in the chest, pointing to a possible leak. In contrast, the Thopaz system offers a graphical display of the air flowing into the system that can be reviewed over the 24‐hour period. When the graph line reaches a 0 flatline graph, no airflow is being detected and no air leakage is suspected (Figure 4B). If no air leaks are detected, the chest tube may be discontinued. Those patients with a failed air leak test should have their chest tubes continued under suction for another 24 hours, with the above tests then repeated. The same holds true for those patients with persistent pneumothorax at 24 hours.
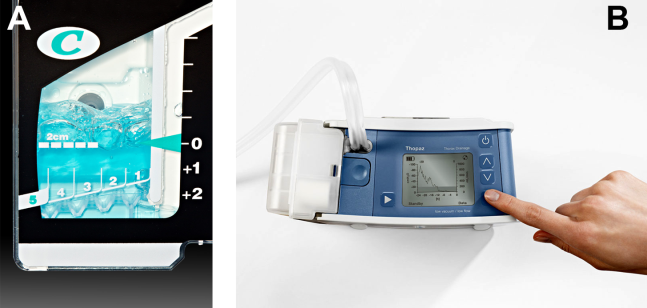
Removal of chest tubes is a simple process that requires the tube to be pulled out of the patient without allowing air to enter the site where the tube was present and where it entered the thorax. Most interventionalists will discontinue the tubes that they have placed. Some small catheters have an internal string that has to be released so the catheter will straighten and pull out easily. Knowledge of the type of catheter or tube that was placed is critical before removal to prevent complications and patient discomfort. Standard chest tubes are straight, smooth plastic and pull out easily but require rapid occlusion of the larger puncture site in the chest wall with an occlusive dressing that often includes petroleum or water‐soluble gel. Some physicians will leave a suture tie when placing the chest tube so that it can be tied down to occlude the site instead of using a dressing.
Consultation of the Cardiothoracic Surgeon or Interventional Pulmonologist
We recommend the involvement of cardiothoracic surgery or interventional pulmonology for patients with nonresolving pneumothorax lasting longer than 48 hours because additional procedures may be necessary. One of the rare but serious complications of a persistent pneumothorax is the formation of a bronchopleural fistula. This communication between the bronchial tree and the pleural space can lead to significant morbidity and mortality. The treatment of a bronchopleural fistula includes medical and surgical options that are beyond the scope of this article but require the expertise of a cardiothoracic surgeon or interventional pulmonologist.[34] Most patients who will not require additional procedures will heal within 48 hours.[11, 35] Decisions regarding more invasive treatment measures can then be made as necessary.[26]
PRACTICAL TIPS
Hospitalists caring for patients with chest tubes are often asked to troubleshoot at the bedside. Scenarios that may be encountered include nonfunctioning tubes, catheter migration, and tube discomfort. Ensuring patency of the tube entails visualizing the tube from the point of entry into the chest wall to the collection chamber and inspecting for kinks or debris clogging the tube. Smaller catheters can be easily kinked during patient positioning and can become clogged. Respiratory variation, which is the movement of the column of fluid in the collection chamber or in the tubing with inspiration and expiration, suggests that the chest tube is patent. This should be part of the daily examination in a patient with a chest tube, and it should also be the first step in assessing sudden dyspnea, hypoxia, pain, or hemodynamic instability. Clogged tubes should be referred to the interventionalists or other physicians who placed them. Chest tubes are typically sutured at the site of entry and securely bandaged to avoid migration but occasionally can be dislodged. This should prompt placement of another tube by an experienced operator. Last, chest tubes can be uncomfortable for patients who may require systemic analgesics. Additionally, tube positioning may ease some of the discomfort. Chest tubes are commonly placed along the midaxillary line and the posterior thorax, leading to discomfort in the recumbent position. Directing the tube anteriorly helps ease some of the discomfort. This can be done using all‐purpose sponges to build a barrier between the skin and the chest tube as it is directed anteriorly. Additional sponges are placed above the tube for extra protection. The gentle curve accomplished by padding the underside of the tube also keeps the tube patent by avoiding sharp kinks as the catheter exits the thorax.
FUTURE TRENDS
Future trends in the management of IP may include shorter duration of tube management (14 hours of suction with air leak evaluation and removal) and the use of even smaller catheters. Outpatient management of IP with small pigtail catheters or small‐caliber tubes in addition to 1‐way valves is also being investigated. The benefits of these practices may include greater patient comfort and lower cost. These approaches will need larger‐scale replication and careful patient selection before they become standard practice.[28, 36]
Ultrasound can be used to assess the presence and size of pneumothoraces that are difficult to visualize by standard chest radiographs. Several studies have established ultrasonography as an effective method of diagnosing pneumothorax and have shown it to have superior sensitivity compared with chest radiography.[1, 18] In the future, the use of ultrasound will likely be more widespread given its performance, portability, ease of use, and relatively low cost.
SUMMARY
IP is a known and costly complication of many medical procedures. The aforementioned algorithms help simplify the management of chest tubes for hospitalists caring for patients with this common complication. This stepwise approach may not only help curtail added expenses related to IPs by decreasing the length of inpatient stays but may also improve patient satisfaction.
Acknowledgments
Disclosure: Mayo does not endorse the products mentioned in this article. The authors report no conflicts of interest.
Box
Clinical Signs and Symptoms of Pneumothorax
Dyspnea
Pleuritic chest pain
Tachypnea
Hypoxia
Decreased breath sounds on affected side
Hyper‐resonant percussion on affected side
Subcutaneous emphysema
A pneumothorax is a collection of air in the space outside the lungs that is trapped within the thorax. This abnormality can occur spontaneously or as the result of trauma. Traumatic pneumothoraces include those resulting from medical interventions such as a transthoracic and transbronchial needle biopsy, central line placement, and positive‐pressure mechanical ventilation. This group is most accurately described as iatrogenic pneumothorax (IP).[1]
IP can be an expected complication of many routine thoracic procedures, but it can also occur accidentally during procedures near the lung or thoracic cavity. Some IPs may be asymptomatic and go undiagnosed, or their diagnosis may be delayed.[2] The majority of iatrogenic pneumothoraces will resolve without complications, and patients will not require medical attention. A small percentage can, however, expand and have the potential to develop into a tension pneumothorax causing severe respiratory distress and mediastinal shift.[3, 4]
The incidence of IP ranges from 0.11% with mechanical ventilation to 2.68% with thoracentesis, according to an analysis of 7.5 million uniform hospital discharge abstracts from 2000.5 A 2010 systematic review of 24 studies that included 6605 patients suggested a 6.0% incidence of pneumothorax following thoracentesis.[3] The highest risk of IP is seen with computed tomography (CT)‐guided lung biopsy, with 1 series of 1098 biopsies showing a 42% incidence; chest tube evacuation was required in 12% of these cases.[4] A Veterans Administration study of patient safety indicators from 2001 to 2004 found that risk‐adjusted rates of IP were increasing over time.[6] It is unclear whether this increase is due to increasing numbers of interventional procedures or to better rates of detection. IP poses a considerable cost to the medical system, with safety studies finding that patients with IP will stay in the hospital approximately 4 days longer and incur an additional $17,000 in charges.[7]
In addition to this financial burden, the lack of consistency in training and guidelines for management of pneumothorax is thought to add to chest tube‐related complications.[8] In 2001, the American College of Chest Physicians (ACCP) published guidelines for the management of spontaneous pneumothorax that do not specifically address IP.[9] In 2010, the British Thoracic Society (BTS) updated their guidelines and included a brief statement on IP that described a higher incidence for it than for spontaneous pneumothorax and noted its relative ease of management.[10] Despite the lack of specific guidelines dedicated to IPs, common clinical practice is to manage iatrogenic defects in a manner similar to that for spontaneous ones. However, studies have shown that the management of pneumothorax remains diverse and that the adherence to these published guidelines is suboptimal.[10, 11] The BTS guidelines favor needle aspiration as the first‐line treatment,[10] whereas the ACCP recommends drainage with catheters over aspiration.[9]
The possibility of this complication, along with the rising rate of invasive interventions being performed, has led to expanded surveillance criteria for IP. Surveillance imaging, clinical observation, or a combination of the 2 may be required, depending on the institution, the risk of the procedure, and the preference of the treating clinician. The algorithms presented here were designed in alignment with both major society guidelines and with the intention of simplifying the treatment regimen for the ease of adoption by hospitalists.
ETIOLOGY AND RISK FACTORS
The etiology and risk factors for IP are multiple, with the most common being interventional‐based procedures. In 535 Veterans Administration patients, the most common precursor procedures were transthoracic needle biopsy (24%), subclavian vein catheterization (22%), thoracentesis (20%), transbronchial biopsy (10%), pleural biopsy (8%), and positive pressure ventilation.[12] IP can also be a rare complication of pacemaker manipulations,[5] and less commonly, bronchoscopy.[13] Patient factors that increase the risk of pneumothorax in the setting of an intervention include age, chronic obstructive lung disease, primary lung cancer, malignant and parapneumonic pleural effusions, empyema, and chronic corticosteroid use.[4] As might be expected, patients with structural lung disease (eg, emphysema with bullae) and poor healing ability (eg, corticosteroid dependent), tend to have IPs more often and to require more complicated interventions for resolution.[14, 15] In some studies, operator experience seems to be inversely related to the rate of IP, and the use of ultrasound is correlated with lower rates of this complication.[1, 3]
PATIENT PRESENTATIONS AND DIAGNOSIS
Clinical signs and symptoms of a significant pneumothorax vary in severity but most often include dyspnea, tachypnea, chest pain, and pleurisy (see Box 1). Post procedure signs or symptoms require further evaluation with imaging, usually a plain chest radiograph. CT can be useful for further evaluation. Small anterior pneumothoraces may be difficult to detect without lateral radiographic imaging or computed tomogram. Ultrasound is being used more frequently at the bedside to make this diagnosis, and various studies of trauma patients have found that it has good sensitivity and specificity.[16, 17] These results have been validated by a recent meta‐analysis comparing ultrasound to chest radiographs for the detection of pneumothorax among trauma, critically ill, and postprocedural patients.[18] This study demonstrated superior sensitivity and similar specificity for ultrasound versus chest radiographs for detection of pneumothorax. More ominous signs, such as tachycardia or hypotension, can be indicative of tension pneumothorax, which requires emergent evacuation.
MANAGEMENT
Once the diagnosis of pneumothorax has been established, treatment options should be guided by defect size and clinical assessment following a defined treatment algorithm (Figure 1). As emphasized by the BTS and ACCP guidelines, we advocate considering the use of symptoms along with defect size to determine the best management course.
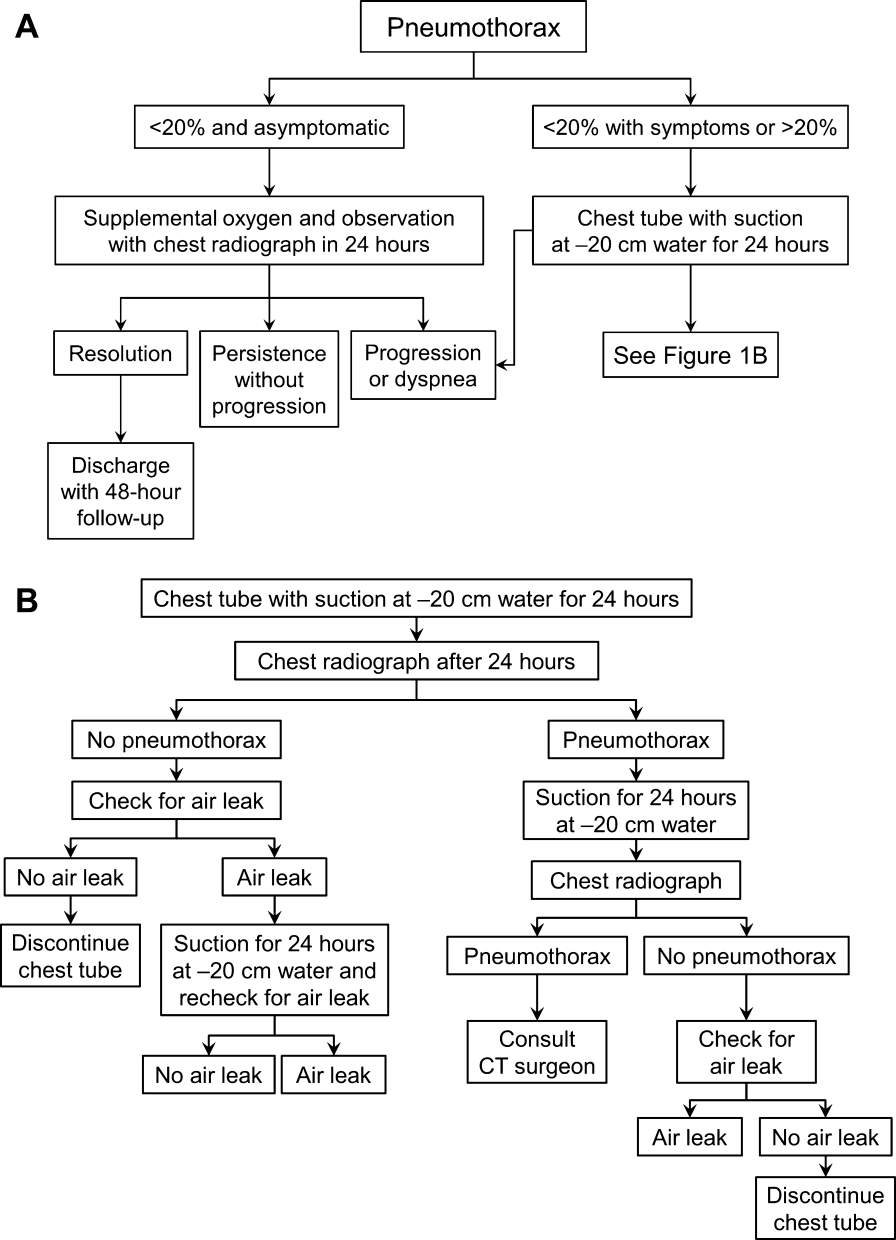
Observation
Defects that involve<20% of the hemithorax in a patient who is clinically asymptomatic and hemodynamically stable can be safely managed by oxygen supplementation and hospital observation. Repeat imaging can be obtained after 12 to 24 hours of defect detection or with symptom change. Patients who display resolution may be discharged home.
Patients who show persistence without progression but are asymptomatic may also be discharged safely, with follow‐up imaging and clinical evaluation 48 hours later.[9, 10] This was demonstrated by Kelly and colleagues,[19] who described the outcomes of 154 patients in a retrospective cohort study. Of the 91 patients treated with outpatient observation, 82 resolved without additional interventions. A recent review article by the same author cites conservative management of small pneumothoraces as being widely accepted.[20] If reimaging shows progression of defect or if the patient becomes more symptomatic, the pneumothorax should be evacuated by 1 of the methods described below.
Aspiration
Aspiration is defined by the ACCP Delphi consensus statement as the removal of pleural air via needle or cannula followed by immediate removal of needle or cannula.[9] This option mandates careful patient selection. It should be considered for small pneumothoraces that cause only mild dyspnea in patients who have no known parenchymal disease. These patients should be observed overnight in the hospital and reimaged 24 hours after aspiration of the pneumothorax. Several authors have reported success with aspiration alone. Yamagami et al.[21] noted the efficacy of manual aspiration immediately after CT‐guided biopsy, with a success rate exceeding 90%. They also noted that evacuated volumes >543 mL correlated with the need for further intervention with a chest tube. This technique is advocated for small pneumothoraces that are recognized shortly after the procedure.
Similarly, Delius and colleagues[22] managed 131 pneumothoraces with aspiration as an alternative to chest tube placement. Of these, 79 were iatrogenic. Aspiration achieved a 75% success rate for all IPs. Small defects defined as <20% of volume had an even higher resolution rate of 87%. Similar findings were demonstrated by Talbot‐Stern et al.[23] in their prospective study of 76 pneumothoraces. Among those that were iatrogenic, 82% resolved after simple aspiration. Faruqi et al.[24] also showed that aspiration is a viable option for IPs. Of the 57 patients with pneumothorax included in their study, 35 were treated with aspiration alone. Iatrogenesis was the culprit in 12 of the 35 manually aspirated cases. Aspiration achieved a success rate of 91.7% in IP. A recent Cochrane database systematic review compared simple aspiration with intercostal tube drainage for primary spontaneous pneumothorax.[25] The authors reported no difference between these methods in terms of success rate, early failure rate, duration of hospital stay, 1‐year success rate, or number of patients who required pleurodesis at 1‐year follow‐up.
Because the algorithms presented in this article were specifically designed for the use by hospitalists, we intentionally omitted aspiration from the decision trees. Most hospitalists would not be expected to evacuate IPs. However, knowledge regarding this option and appropriate follow‐up are valuable to internists, because many interventionalists admit patients to the hospital service for overnight observation. An asymptomatic postaspiration patient, who on subsequent imaging demonstrates resolution or persistence without progression of pneumothorax, may be discharged with 48‐hour follow‐up.
Placement of Catheter or Chest Tube Drainage
Most patients with a clinically significant pneumothorax will require evacuation of the air. Pneumothoraces larger than 20% or that produce symptoms warrant chest tube management and inpatient observation (Figure 1B). Traditionally, large tubes with 20 cm of water on continuous suction are used and have been studied the most widely. Several authors have shown that smaller tubes can effectively drain a pneumothorax.[26, 27, 28, 29] Small‐bore catheters (8F14F), which can be inserted percutaneously, have been shown to provide effective lung re‐expansion with minimal morbidity[8] and may be better tolerated by patients with uncomplicated pneumothoraces (Figure 2). Terzi and colleagues[30] have shown that smaller tubes cause less discomfort to patients at rest, with cough, and at the time of tube removal.
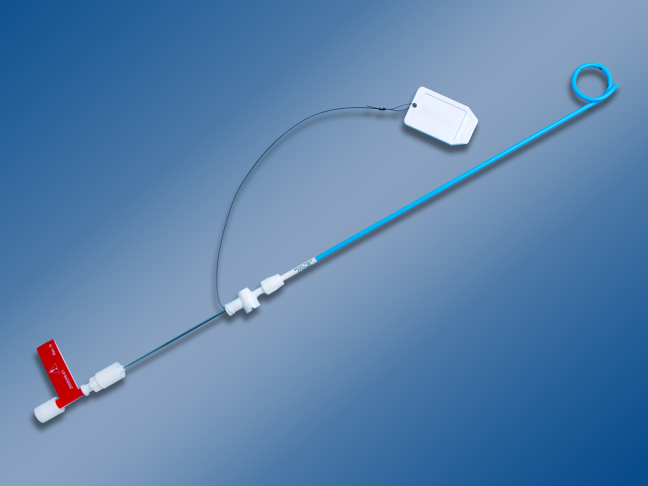
At most US institutions, catheters and chest tubes are connected to all‐purpose drainage systems. Although commercially available through a variety of manufacturers, they share similar design principles because they replicate the 3‐bottle system described in detail elsewhere in the literature.[31] We have limited our discussion to 3 pleural evacuation systems because it is our intention to familiarize hospitalists with the units that they are most likely to encounter. The first 2 systems have been studied and described by Baumann and colleagues[32] as being commonplace and reasonably reliable. These include the Oasis (Atrium Medical Corp., Hudson, NH) (Figure 3A) and the Pleur‐evac (Teleflex Inc., Limerick, PA). The third unit is the Thopaz digital thoracic drainage system (Medela Inc., McHenry, IL) (Figure 3B). The Thopaz is unique in its inclusion of a suction source and digital capability. Although it utilizes the same principles of all pleural evacuation devices, its setup and information output require that one be familiar with its digital format.
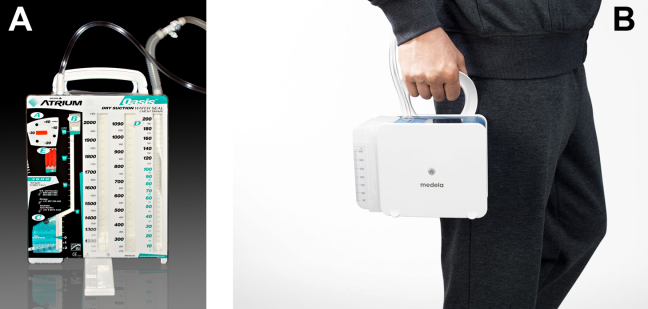
Suction Versus Water Seal
The chest tube should be placed initially to a suction pressure level of 20 cm of water for 24 hours to maximize lung expansion and evacuate all extrapulmonary air. Suction pressure is set on the Pleur‐evac and Atrium drainage systems by a manual dial that reads to a water pressure of 0 to 40 cm. The default setting from the manufacturer is 20 cm of water. This level of suction is present only when the drainage system is connected to a wall or a portable suction device. The only confirmation of suction presence in the Atrium system is the deployment of the orange bellows (located under the dial) to the level of the arrow tip (Figure 3A). The Pleur‐evac system has a red stripe along the circular edge of the dial that appears at the set level of suction when negative pressure is being applied. It is important to be aware that when patients are disconnected from the wall or the portable suction apparatus, they are on water seal or gravity. These terms are synonymous with no suction. On the Thopaz, a digital menu directs operation, and levels of suction can be selected from water seal (no suction) up to 40 cm of water. We recommend using suction to 20 cm of water given the scarce evidence supporting higher levels of negative pressure. Some clinicians prefer placing patients on water seal for some time before moving toward tube discontinuance, but this is a matter of preference, and no substantial evidence exists to show that any 1 method is superior.[8, 33]
Assessing for Air Leak
If there is improvement or resolution of the pneumothorax after 24 hours, the presence of an air leak should be assessed; if no leak is present, the chest tube can be safely removed. In the context of chest tubes, the term air leak refers to residual air between the lung and the chest wall. It is possible to see resolution of a pneumothorax on chest radiographs and still have an air leak. This situation is created by a perfect balance between the pleural air evacuation by the catheter and the flow of air exiting from the lung puncture. This would result in reaccumulation of the pneumothorax if the chest tube is removed prematurely. It should also be kept in mind that chest radiographs may miss a small pneumothorax given their relatively low sensitivity.[18] Therefore, the absence of an air leak needs to be documented before the chest tube is discontinued. Depending on the type of drainage system (Atrium, Pleur‐evac, or Thopaz), this assessment can be done in several ways. All systems can be assessed for air leak by clamping the actual chest tube for 2 to 4 hours and then repeating the chest radiograph. Clamping a chest tube simulates the condition of not having a chest tube. Chest tubes should never be clamped without supervision and only with the knowledge of nursing personnel. The onset of chest pain or dyspnea in a patient with a clamped tube mandates immediate removal of the clamp and a return to suction. A repeat chest radiograph showing reaccumulation or expansion of the pneumothorax after clamping indicates that the air leak has not resolved and the chest tube must remain in place and returned to suction. Simpler and more time‐efficient methods of detecting air leaks are available with both cardiothoracic drainage systems.
For the Atrium and Pleur‐evac models, there is a graded panel through which one can visualize air leaks being funneled through water (Figure 4A). Having the patient cough several times or perform a Valsalva maneuver should release any air trapped within the chest into this chamber, where bubbles can be visualized as they travel through the water. The presence of bubbles indicates the presence of residual air in the chest, pointing to a possible leak. In contrast, the Thopaz system offers a graphical display of the air flowing into the system that can be reviewed over the 24‐hour period. When the graph line reaches a 0 flatline graph, no airflow is being detected and no air leakage is suspected (Figure 4B). If no air leaks are detected, the chest tube may be discontinued. Those patients with a failed air leak test should have their chest tubes continued under suction for another 24 hours, with the above tests then repeated. The same holds true for those patients with persistent pneumothorax at 24 hours.
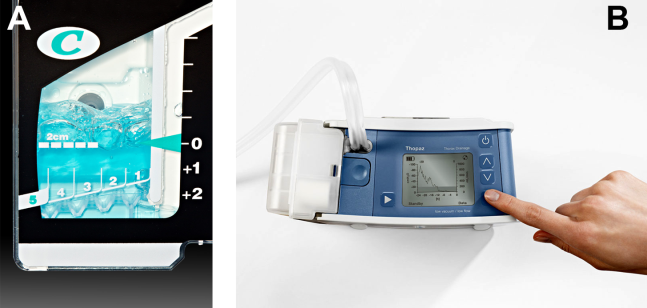
Removal of chest tubes is a simple process that requires the tube to be pulled out of the patient without allowing air to enter the site where the tube was present and where it entered the thorax. Most interventionalists will discontinue the tubes that they have placed. Some small catheters have an internal string that has to be released so the catheter will straighten and pull out easily. Knowledge of the type of catheter or tube that was placed is critical before removal to prevent complications and patient discomfort. Standard chest tubes are straight, smooth plastic and pull out easily but require rapid occlusion of the larger puncture site in the chest wall with an occlusive dressing that often includes petroleum or water‐soluble gel. Some physicians will leave a suture tie when placing the chest tube so that it can be tied down to occlude the site instead of using a dressing.
Consultation of the Cardiothoracic Surgeon or Interventional Pulmonologist
We recommend the involvement of cardiothoracic surgery or interventional pulmonology for patients with nonresolving pneumothorax lasting longer than 48 hours because additional procedures may be necessary. One of the rare but serious complications of a persistent pneumothorax is the formation of a bronchopleural fistula. This communication between the bronchial tree and the pleural space can lead to significant morbidity and mortality. The treatment of a bronchopleural fistula includes medical and surgical options that are beyond the scope of this article but require the expertise of a cardiothoracic surgeon or interventional pulmonologist.[34] Most patients who will not require additional procedures will heal within 48 hours.[11, 35] Decisions regarding more invasive treatment measures can then be made as necessary.[26]
PRACTICAL TIPS
Hospitalists caring for patients with chest tubes are often asked to troubleshoot at the bedside. Scenarios that may be encountered include nonfunctioning tubes, catheter migration, and tube discomfort. Ensuring patency of the tube entails visualizing the tube from the point of entry into the chest wall to the collection chamber and inspecting for kinks or debris clogging the tube. Smaller catheters can be easily kinked during patient positioning and can become clogged. Respiratory variation, which is the movement of the column of fluid in the collection chamber or in the tubing with inspiration and expiration, suggests that the chest tube is patent. This should be part of the daily examination in a patient with a chest tube, and it should also be the first step in assessing sudden dyspnea, hypoxia, pain, or hemodynamic instability. Clogged tubes should be referred to the interventionalists or other physicians who placed them. Chest tubes are typically sutured at the site of entry and securely bandaged to avoid migration but occasionally can be dislodged. This should prompt placement of another tube by an experienced operator. Last, chest tubes can be uncomfortable for patients who may require systemic analgesics. Additionally, tube positioning may ease some of the discomfort. Chest tubes are commonly placed along the midaxillary line and the posterior thorax, leading to discomfort in the recumbent position. Directing the tube anteriorly helps ease some of the discomfort. This can be done using all‐purpose sponges to build a barrier between the skin and the chest tube as it is directed anteriorly. Additional sponges are placed above the tube for extra protection. The gentle curve accomplished by padding the underside of the tube also keeps the tube patent by avoiding sharp kinks as the catheter exits the thorax.
FUTURE TRENDS
Future trends in the management of IP may include shorter duration of tube management (14 hours of suction with air leak evaluation and removal) and the use of even smaller catheters. Outpatient management of IP with small pigtail catheters or small‐caliber tubes in addition to 1‐way valves is also being investigated. The benefits of these practices may include greater patient comfort and lower cost. These approaches will need larger‐scale replication and careful patient selection before they become standard practice.[28, 36]
Ultrasound can be used to assess the presence and size of pneumothoraces that are difficult to visualize by standard chest radiographs. Several studies have established ultrasonography as an effective method of diagnosing pneumothorax and have shown it to have superior sensitivity compared with chest radiography.[1, 18] In the future, the use of ultrasound will likely be more widespread given its performance, portability, ease of use, and relatively low cost.
SUMMARY
IP is a known and costly complication of many medical procedures. The aforementioned algorithms help simplify the management of chest tubes for hospitalists caring for patients with this common complication. This stepwise approach may not only help curtail added expenses related to IPs by decreasing the length of inpatient stays but may also improve patient satisfaction.
Acknowledgments
Disclosure: Mayo does not endorse the products mentioned in this article. The authors report no conflicts of interest.
Box
Clinical Signs and Symptoms of Pneumothorax
Dyspnea
Pleuritic chest pain
Tachypnea
Hypoxia
Decreased breath sounds on affected side
Hyper‐resonant percussion on affected side
Subcutaneous emphysema
- Management of pneumothorax. Semin Respir Crit Care Med. 2010;31(6):769–780. , .
- Pneumothorax. Respirology. 2004;9(2):157–164. , .
- Pneumothorax following thoracentesis: a systematic review and meta‐analysis. Arch Intern Med. 2010;170(4):332–339. , , , .
- Incidence of and risk factors for pneumothorax and chest tube placement after CT fluoroscopy‐guided percutaneous lung biopsy: retrospective analysis of the procedures conducted over a 9‐year period. AJR Am J Roentgenol. 2010;194(3):809–814. , , , et al.
- Accidental iatrogenic pneumothorax in hospitalized patients. Med Care. 2006;44(2):182–186. , , .
- Tracking rates of patient safety indicators over time: lessons from the Veterans Administration. Med Care. 2006;44(9):850–861. , , , et al.
- Excess length of stay, charges, and mortality attributable to medical injuries during hospitalization. JAMA. 2003;290(14):1868–1874. , .
- Preliminary report of a prospective, randomized trial of underwater seal for spontaneous and iatrogenic pneumothorax. J Am Coll Surg. 2007;204(1):84–90. , , , , .
- AACP Pneumothorax Consensus Group. Management of spontaneous pneumothorax: an American College of Chest Physicians Delphi consensus statement. Chest. 2001;119(2):590–602. , , , et al.;
- BTS Pleural Disease Guideline Group. Management of spontaneous pneumothorax: British Thoracic Society Pleural Disease Guideline 2010. Thorax. 2010;65(suppl 2):ii18–ii31. , , ;
- The clinician's perspective on pneumothorax management. Chest. 1997;112(3):822–828. , .
- Iatrogenic pneumothorax: etiology and morbidity: results of a Department of Veterans Affairs Cooperative Study. Respiration. 1992;59(4):215–220. , , , .
- Severe complications of bronchoscopy. Respiration. 2008;76(4):429–433. , , , , , .
- Incidence and risk factors of delayed pneumothorax after transthoracic needle biopsy of the lung. Chest. 2004;126(5):1516–1521. , , , et al.
- Factors associated with pneumothorax and pneumothorax requiring treatment after percutaneous lung biopsy in 443 consecutive patients. J Vasc Interv Radiol. 2004;15(5):479–483. , , , , , .
- Ultrasound detection of pneumothorax compared with chest X‐ray and computed tomography scan. Am Surg. 2011;77(4):480–484. , .
- Sensitivity of bedside ultrasound and supine anteroposterior chest radiographs for the identification of pneumothorax after blunt trauma. Acad Emerg Med. 2010;17(1):11–17. , .
- Diagnosis of pneumothorax by radiography and ultrasonography: a meta‐analysis. Chest. 2011;140(4):859–866. , , , , .
- Outcomes of emergency department patients treated for primary spontaneous pneumothorax. Chest. 2008;134(5):1033–1036. , , .
- Review of management of primary spontaneous pneumothorax: is the best evidence clearer 15 years on? Emerg Med Australas. 2007;19(4):303–308. .
- Efficacy of manual aspiration immediately after complicated pneumothorax in CT‐guided lung biopsy. J Vasc Interv Radiol. 2005;16(4):477–483. , , , , , .
- Catheter aspiration for simple pneumothorax: experience with 114 patients. Arch Surg. 1998;124(7):833–836. , , , , , .
- Catheter aspiration for simple pneumothorax. J Emerg Med. 1986;4(6):437–442. , , , , .
- Role of simple needle aspiration in the management of pneumothorax. Indian J Chest Dis Allied Sci. 2004;46(3):183–190. , , , .
- Simple aspiration versus intercostal tube drainage for primary spontaneous pneumothorax in adults. Cochrane Database Syst Rev. 2007;(1):CD004479. , , .
- Evaluation of conventional chest tube therapy for iatrogenic pneumothorax. Chest. 1993;104(6):1770–1772. , , , .
- Outpatient treatment of iatrogenic pneumothorax after needle biopsy. Radiology. 1997;205(1):249–252. , , , .
- Outpatient management of postbiopsy pneumothorax with small‐caliber chest tubes: factors affecting the need for prolonged drainage and additional interventions. Cardiovasc Intervent Radiol. 2008;31(2):342–348. , , , , , .
- Management of primary and secondary pneumothorax using a small‐bore thoracic catheter. Interact Cardiovasc Thorac Surg. 2010;11(2):146–149. , .
- The use of flexible spiral drains after non‐cardiac thoracic surgery: a clinical study. Eur J Cardiothorac Surg. 2005;27(1):134–137. , , , et al.
- Pleural Diseases. 5th ed. Philadelphia: PA: Lippincott Williams 2007. .
- Comparison of function of commercially available pleural drainage units and catheters. Chest. 2003;123(6):1878–1886. , , , .
- Catheter drainage of spontaneous pneumothorax: suction or no suction, early or late removal? Thorax. 1982;37(1):46–48. , .
- Bronchopleural fistulas: an overview of the problem with special focus on endoscopic management. Chest. 2005;128(6):3955–3965. , .
- Timing of invasive procedures in therapy for primary and secondary spontaneous pneumothorax. Arch Surg. 1991;126(6):764–766. , , , .
- A treatment algorithm for pneumothoraces complicating central venous catheter insertion. Am J Surg. 2000;180(6):523–526. , , , , .
- Management of pneumothorax. Semin Respir Crit Care Med. 2010;31(6):769–780. , .
- Pneumothorax. Respirology. 2004;9(2):157–164. , .
- Pneumothorax following thoracentesis: a systematic review and meta‐analysis. Arch Intern Med. 2010;170(4):332–339. , , , .
- Incidence of and risk factors for pneumothorax and chest tube placement after CT fluoroscopy‐guided percutaneous lung biopsy: retrospective analysis of the procedures conducted over a 9‐year period. AJR Am J Roentgenol. 2010;194(3):809–814. , , , et al.
- Accidental iatrogenic pneumothorax in hospitalized patients. Med Care. 2006;44(2):182–186. , , .
- Tracking rates of patient safety indicators over time: lessons from the Veterans Administration. Med Care. 2006;44(9):850–861. , , , et al.
- Excess length of stay, charges, and mortality attributable to medical injuries during hospitalization. JAMA. 2003;290(14):1868–1874. , .
- Preliminary report of a prospective, randomized trial of underwater seal for spontaneous and iatrogenic pneumothorax. J Am Coll Surg. 2007;204(1):84–90. , , , , .
- AACP Pneumothorax Consensus Group. Management of spontaneous pneumothorax: an American College of Chest Physicians Delphi consensus statement. Chest. 2001;119(2):590–602. , , , et al.;
- BTS Pleural Disease Guideline Group. Management of spontaneous pneumothorax: British Thoracic Society Pleural Disease Guideline 2010. Thorax. 2010;65(suppl 2):ii18–ii31. , , ;
- The clinician's perspective on pneumothorax management. Chest. 1997;112(3):822–828. , .
- Iatrogenic pneumothorax: etiology and morbidity: results of a Department of Veterans Affairs Cooperative Study. Respiration. 1992;59(4):215–220. , , , .
- Severe complications of bronchoscopy. Respiration. 2008;76(4):429–433. , , , , , .
- Incidence and risk factors of delayed pneumothorax after transthoracic needle biopsy of the lung. Chest. 2004;126(5):1516–1521. , , , et al.
- Factors associated with pneumothorax and pneumothorax requiring treatment after percutaneous lung biopsy in 443 consecutive patients. J Vasc Interv Radiol. 2004;15(5):479–483. , , , , , .
- Ultrasound detection of pneumothorax compared with chest X‐ray and computed tomography scan. Am Surg. 2011;77(4):480–484. , .
- Sensitivity of bedside ultrasound and supine anteroposterior chest radiographs for the identification of pneumothorax after blunt trauma. Acad Emerg Med. 2010;17(1):11–17. , .
- Diagnosis of pneumothorax by radiography and ultrasonography: a meta‐analysis. Chest. 2011;140(4):859–866. , , , , .
- Outcomes of emergency department patients treated for primary spontaneous pneumothorax. Chest. 2008;134(5):1033–1036. , , .
- Review of management of primary spontaneous pneumothorax: is the best evidence clearer 15 years on? Emerg Med Australas. 2007;19(4):303–308. .
- Efficacy of manual aspiration immediately after complicated pneumothorax in CT‐guided lung biopsy. J Vasc Interv Radiol. 2005;16(4):477–483. , , , , , .
- Catheter aspiration for simple pneumothorax: experience with 114 patients. Arch Surg. 1998;124(7):833–836. , , , , , .
- Catheter aspiration for simple pneumothorax. J Emerg Med. 1986;4(6):437–442. , , , , .
- Role of simple needle aspiration in the management of pneumothorax. Indian J Chest Dis Allied Sci. 2004;46(3):183–190. , , , .
- Simple aspiration versus intercostal tube drainage for primary spontaneous pneumothorax in adults. Cochrane Database Syst Rev. 2007;(1):CD004479. , , .
- Evaluation of conventional chest tube therapy for iatrogenic pneumothorax. Chest. 1993;104(6):1770–1772. , , , .
- Outpatient treatment of iatrogenic pneumothorax after needle biopsy. Radiology. 1997;205(1):249–252. , , , .
- Outpatient management of postbiopsy pneumothorax with small‐caliber chest tubes: factors affecting the need for prolonged drainage and additional interventions. Cardiovasc Intervent Radiol. 2008;31(2):342–348. , , , , , .
- Management of primary and secondary pneumothorax using a small‐bore thoracic catheter. Interact Cardiovasc Thorac Surg. 2010;11(2):146–149. , .
- The use of flexible spiral drains after non‐cardiac thoracic surgery: a clinical study. Eur J Cardiothorac Surg. 2005;27(1):134–137. , , , et al.
- Pleural Diseases. 5th ed. Philadelphia: PA: Lippincott Williams 2007. .
- Comparison of function of commercially available pleural drainage units and catheters. Chest. 2003;123(6):1878–1886. , , , .
- Catheter drainage of spontaneous pneumothorax: suction or no suction, early or late removal? Thorax. 1982;37(1):46–48. , .
- Bronchopleural fistulas: an overview of the problem with special focus on endoscopic management. Chest. 2005;128(6):3955–3965. , .
- Timing of invasive procedures in therapy for primary and secondary spontaneous pneumothorax. Arch Surg. 1991;126(6):764–766. , , , .
- A treatment algorithm for pneumothoraces complicating central venous catheter insertion. Am J Surg. 2000;180(6):523–526. , , , , .