User login
Bronchiolitis and Discharge Criteria
Although bronchiolitis is the leading cause of hospitalization for US infants,[1] there is a lack of basic prospective data about the expected inpatient clinical course and ongoing uncertainty about when a hospitalized child is ready for discharge to home.[2] This lack of data about children's readiness for discharge may result in variable hospital length‐of‐stay (LOS).[3, 4, 5]
One specific source of variability in discharge readiness and LOS variability may be the lack of consensus about safe threshold oxygen saturation values for discharge in children hospitalized with bronchiolitis.[6, 7] In 2006, the Scottish Intercollegiate Guidelines Network recommended a discharge room air oxygen (RAO2) saturation threshold of 95%.[8] The same year, the American Academy of Pediatrics (AAP) bronchiolitis clinical practice guideline stated that oxygen is not needed for children with RAO2 saturations 90% who are feeding well and have minimal respiratory distress.[9] There is a need for prospective studies to help clinicians make evidenced‐based discharge decisions for this common condition.
We performed a prospective, multicenter, multiyear study[10, 11, 12] to examine the typical inpatient clinical course of and to develop hospital discharge guidelines for children age <2 years hospitalized with bronchiolitis. We hypothesized that children would not worsen clinically and would be safe to discharge home once their respiratory status improved and they were able to remain hydrated.
METHODS
Study Design and Population
We conducted a prospective, multicenter cohort study for 3 consecutive years during the 2007 to 2010 winter seasons, as part of the Multicenter Airway Research Collaboration (MARC), a program of the Emergency Medicine Network (
All patients were treated at the discretion of the treating physician. Inclusion criteria were an attending physician's diagnosis of bronchiolitis, age <2 years, and the ability of the parent/guardian to give informed consent. The exclusion criteria were previous enrollment and transfer to a participating hospital >48 hours after the original admission time. Therefore, children with comorbid conditions were included in this study. All consent and data forms were translated into Spanish. The institutional review board at each of the 16 participating hospitals approved the study.
Of the 2207 enrolled children, we excluded 109 (5%) children with a hospital LOS <1 day due to inadequate time to capture the required data for the present analysis. Among the 2098 remaining children, 1916 (91%) had daily inpatient data on all factors used to define clinical improvement and clinical worsening. Thus, the analytic cohort was comprised of 1916 children hospitalized for bronchiolitis.
Data Collection
Investigators conducted detailed structured interviews. Chart reviews were conducted to obtain preadmission and daily hospital clinical data including respiratory rates, daily respiratory rate trends, degree of retractions, oxygen saturation, daily oxygen saturation trends, medical management, and disposition. These data were manually reviewed, and site investigators were queried about missing data and discrepancies. A follow‐up telephone interview was conducted with families 1 week after discharge to examine relapse events at both 24 hours and 7 days.
We used the question: How long ago did the following symptoms [eg, difficulty breathing] begin [for the] current illness? to estimate the onset of the current illness. Pulse was categorized as low, normal, or high based on age‐related heart rate values.[13] Presence of apnea was recorded daily by site investigators.[14]
Nasopharyngeal Aspirate Collection and Virology Testing
As described previously, site teams used a standardized protocol to collect nasopharyngeal aspirates,[11] which were tested for respiratory syncytial virus (RSV) types A and B; rhinovirus (RV); parainfluenza virus types 1, 2, and 3; influenza virus types A and B; 2009 novel H1N1; human metapneumovirus; coronaviruses NL‐63, HKU1, OC43, and 229E; enterovirus, and adenovirus using polymerase chain reaction.[11, 15, 16, 17]
Defining Clinical Improvement and Worsening
Clinical improvement criteria were based on the 2006 AAP guidelines.[9] For respiratory rate and oxygen saturation, clinicians estimated average daily respiratory rate and oxygen saturation based on the recorded readings from the previous 24 hours. This estimation reflects the process clinicians use when rounding on their hospitalized patients, and thus may be more similar to standard clinical practice than a calculated mean. The respiratory rate criteria are adjusted for age.[18, 19] For daily estimated average oxygen saturation we used the AAP criteria of RAO2 saturation of 90%. Considering that oxygen saturation is the main determinant of LOS,[20] healthy infants age <6 months may have transient oxygen saturations of around 80%,[21] and that errors in estimation may occur, we included a lowest RAO2 of 88% in our improvement criteria. By combining the dichotomized estimated oxygen saturation (90% or not) with the lower limit of 88%, there was little room for erroneous conclusions. A child was considered clinically improved on the earliest date he/she met all of the following criteria: (1) none or mild retractions and improved or stable retractions compared with the previous inpatient day; (2) daily estimated average respiratory rate (RR) <60 breaths per minute for age <6 months, <55 breaths/minute for age 6 to 11 months, and <45 breaths/minute for age 12 months with a decreasing or stable trend over the course of the current day; (3) daily estimated average RAO2 saturation 90%, lowest RAO2 saturation 88%[21]; and (4) not receiving intravenous (IV) fluids or for children receiving IV fluids a clinician report of the child maintaining oral hydration. Children who reached the clinical improvement criteria were considered clinically worse if they required intensive care or had the inverse of 1 of the improvement criteria: moderate/severe retractions that were worse compared with the previous inpatient day, daily average RR 60 with an increasing trend over the current day, need for oxygen, or need for IV fluids.
Statistical Analyses
All analyses were performed using Stata 12.0 (StataCorp, College Station, TX). Data are presented as proportions with 95% confidence intervals (95% CIs), means with standard deviations, and medians with interquartile ranges (IQR). To examine potential factors associated with clinical worsening after reaching clinical improvement, we used 2, Fisher exact, Student t test, and Kruskall‐Wallis tests, as appropriate.
Adjusted analyses used generalized linear mixed models with a logit link to identify independent risk factors for worsening after reaching clinical improvement. Fixed effects for patient‐level factors and a random site effect were used. Factors were tested for inclusion in the multivariable model if they were found to be associated with worsening in unadjusted analyses (P<0.20) or were considered clinically important. Results are reported as odds ratios with 95% CIs.
We performed several sensitivity analyses to evaluate these improvement criteria: (1) we excluded the lowest RAO2 saturation requirement of 88%, (2) we examined a 94% daily estimated average RAO2 saturation threshold,[22] (3) we examined a 95% daily estimated average RAO2 saturation threshold,[8] and (4) we examined children age <12 months with no history of wheeze.
RESULTS
There were 1916 children hospitalized with bronchiolitis with data on all factors used to define clinical improvement and clinical worsening. The median number of days from the beginning of difficulty breathing until admission was 2 days (IQR, 15.5 days; range, 18 days) and from the beginning of difficulty breathing until clinical improvement was 4 days (IQR, 37.5 days; range, 133 days) (Figure 1). The variance for days to admission was significantly less than the variance for days to clinical improvement (P<0.001).
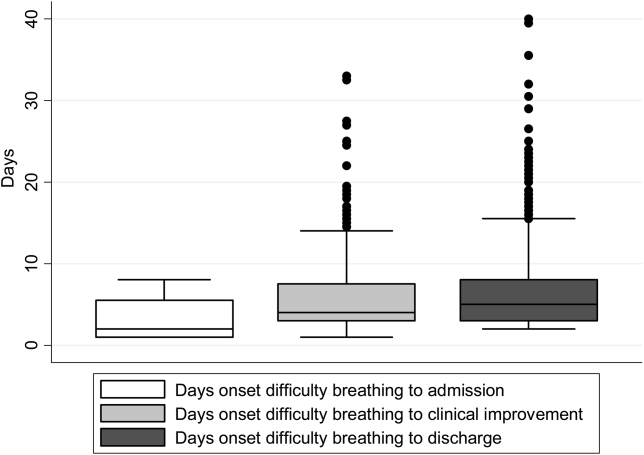
In this observational study, clinicians discharged 214 (11%) of the 1916 children before meeting the definition of clinical improvement. Thus, 1702 (89%; 95% CI: 87%‐90%) children reached the clinical improvement criteria, had a LOS >1 day, and had data on all factors (Figure 2).
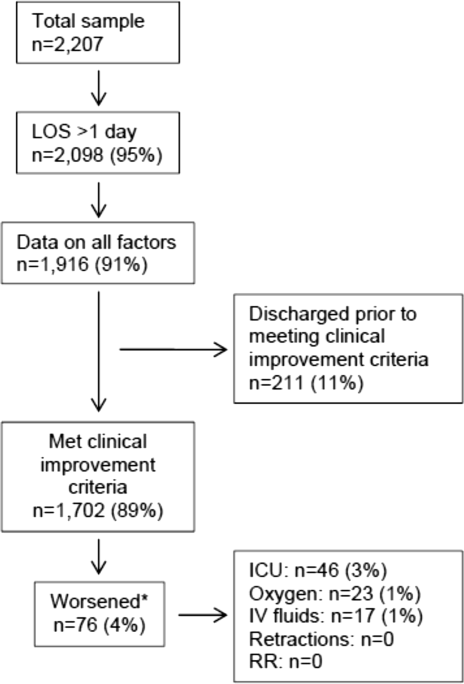
Of the 1702 children who met the clinical improvement criteria, there were 76 children (4%; 95% CI: 3%5%) who worsened (Figure 2). The worsening occurred within a median of 1 day (IQR, 13 days) of clinical improvement. Forty‐six (3%) of the children required transfer to the ICU (1 required intubation, 1 required continuous positive airway pressure, and 4 had apnea), 23 (1%) required oxygen, and 17 (1%) required IV fluids. Eight percent of children met multiple criteria for worsening. A comparison between children who did and did not worsen is shown in Table 1. In general, children who worsened after improvement were younger and born earlier. These children also presented in more severe respiratory distress, had moderate or severe retractions, oxygen saturation <85% at hospitalization, inadequate oral intake, and apnea documented during the hospitalization. Neither viral etiology nor site of care influenced whether the children worsened after improving. However, stratified analysis of children based on initial location of admission (ie, ICU or ward) showed that among the children admitted to the ICU from the emergency department (ED), 89% met the improvement criteria and 19% clinically worsened. In contrast, among children admitted to the ward from the ED, 89% met the improvement criteria, and only 2% clinically worsened. Stratified multivariable models based on the initial location of admission from the ED (ie, ICU or ward) were not possible due to small sample sizes after stratification. None of these children had relapse events requiring rehospitalization within either 24 hours or 7 days of discharge.
Did Not Worsen, n=1,626 | Worsened, n=76 | P Value | |
---|---|---|---|
| |||
Demographic characteristics | |||
Age <2 months, % | 29 | 57 | <0.001 |
Month of birth, % | 0.02 | ||
OctoberMarch | 61 | 75 | |
AprilSeptember | 39 | 25 | |
Sex, % | 0.51 | ||
Male | 59 | 55 | |
Female | 41 | 45 | |
Race, % | 0.050 | ||
White | 63 | 58 | |
Black | 23 | 34 | |
Other or missing | 14 | 8 | |
Hispanic ethnicity, % | 37 | 22 | 0.01 |
Insurance, % | 0.87 | ||
Nonprivate | 68 | 67 | |
Private | 32 | 33 | |
Medical history | |||
Gestational age <37 weeks, % | 23 | 39 | 0.002 |
Birth weight, % | 0.52 | ||
<5 lbs | 13 | 12 | |
5 lbs | 34 | 41 | |
7 lbs | 53 | 47 | |
Mother's age, median (IQR) | 27 (2333) | 27 (2233) | 0.54 |
Is or was breastfed, % | 61 | 51 | 0.10 |
Smoked during pregnancy, % | 15 | 20 | 0.22 |
Exposure to smoke, % | 13 | 20 | 0.11 |
Family history of asthma, % | 0.89 | ||
Neither parent | 68 | 64 | |
Either mother or father | 27 | 30 | |
Both parents | 4 | 4 | |
Do not know/missing | 2 | 1 | |
History of wheezing, % | 23 | 17 | 0.24 |
History of eczema, % | 16 | 7 | 0.04 |
History of intubation, % | 9 | 12 | 0.50 |
Major, relevant, comorbid medical disorder, % | 20 | 24 | 0.46 |
Current illness | |||
When difficulty breathing began, preadmission, % | 0.63 | ||
1 day | 70 | 75 | |
<1 day | 28 | 23 | |
No difficulty preadmission | 2 | 3 | |
Weight, lbs, median (IQR) | 12.3 (8.817.4) | 9.0 (6.613.2) | 0.001 |
Temperature, F, median (IQR) | 99.5 (98.6100.6) | 99.4 (98.1100.4) | 0.06 |
Pulse, beats per minute by age | 0.82 | ||
Low | 0.3 | 0 | |
Normal | 48 | 46 | |
High | 51 | 54 | |
Respiratory rate, breaths per minute, median (IQR) | 48 (4060) | 48 (3864) | 0.28 |
Retractions, % | 0.001 | ||
None | 22 | 25 | |
Mild | 43 | 24 | |
Moderate | 26 | 33 | |
Severe | 4 | 12 | |
Missing | 5 | 7 | |
Oxygen saturation by pulse oximetry or ABG, % | 0.001 | ||
<85 | 4 | 12 | |
8587.9 | 3 | 4 | |
8889.9 | 5 | 0 | |
9093.9 | 18 | 11 | |
94 | 72 | 73 | |
Oral intake, % | <0.001 | ||
Adequate | 45 | 22 | |
Inadequate | 42 | 63 | |
Missing | 13 | 14 | |
Presence of apnea, % | 7 | 24 | <0.001 |
RSV‐A, % | 44 | 41 | 0.54 |
RSV‐B, % | 30 | 25 | 0.36 |
HRV, % | 24 | 24 | 0.88 |
Chest x‐ray results during ED/preadmission visit | |||
Atelectasis | 12 | 13 | 0.77 |
Infiltrate | 13 | 11 | 0.50 |
Hyperinflated | 18 | 21 | 0.47 |
Peribronchial cuffing/thickening | 23 | 17 | 0.32 |
Normal | 14 | 16 | 0.75 |
White blood count, median (IQR) | 11.2 (8.714.4) | 11.9 (9.214.4) | 0.60 |
Platelet count, median (IQR) | 395 (317490) | 430 (299537) | 0.56 |
Sodium, median (IQR) | 138 (136140) | 137 (135138) | 0.19 |
Hospital length of stay, median (IQR) | 2 (14) | 4.5 (28) | <0.001 |
One‐week follow‐up | |||
Relapse within 24 hours of hospital discharge requiring hospital admission, % | 0.5 | 0 | 0.56 |
Relapse within 7 days of hospital discharge requiring hospital admission, % | 1 | 0 | 0.35 |
On multivariable analysis (Table 2), independent risk factors for worsening after reaching the clinical improvement criteria were young age, preterm birth, and presenting to care with more severe bronchiolitis represented by severe retractions, inadequate oral intake, or apnea. To further evaluate the improvement criteria in the current analysis, multiple sensitivity analyses were conducted. The frequency of clinical worsening after reaching the improvement criteria was stable when we examined different RA02 criteria in sensitivity analyses: (1) excluding RA02 as a criterion for improvement: 90% met improvement criteria and 4% experienced clinical worsening, (2) changing the average RA02 threshold for clinical improvement to 94%: 62% met improvement criteria and 6% experienced clinical worsening, and (3) changing the average RA02 threshold for clinical improvement to 95%: 47% met improvement criteria and 5% experienced clinical worsening. Furthermore, stratifying by age <2 months and restricting to more stringent definitions of bronchiolitis (ie, age <1 year or age <1 year+no history of wheezing) also did not materially change the results (see Supporting Figure 1 in the online version of this article).
Odds Ratio | 95% CI | P Value | |
---|---|---|---|
| |||
Age <2 months | 3.51 | 2.07‐5.94 | <0.001 |
Gestational age <37 weeks | 1.94 | 1.13‐3.32 | 0.02 |
Retractions | |||
None | 1.30 | 0.80‐3.23 | 0.19 |
Mild | 1.0 | Reference | |
Moderate | 1.91 | 0.99‐3.71 | 0.06 |
Severe | 5.55 | 2.1214.50 | <0.001 |
Missing | 1.70 | 0.53‐5.42 | 0.37 |
Oral intake | |||
Adequate | 1.00 | Reference | |
Inadequate | 2.54 | 1.39‐4.62 | 0.002 |
Unknown/missing | 1.88 | 0.79‐4.44 | 0.15 |
Presence of apnea | 2.87 | 1.45‐5.68 | 0.003 |
We compared the 214 children who were discharged prior to reaching clinical improvement with the 1702 children who reached the clinical improvement criteria. The 214 children were less likely to be age <2 months (22% vs 30%; P=0.02). These 2 groups (214 vs 1702) were similar with respect to severe retractions (2% vs 4%; P=0.13), median respiratory rate (48 vs 48; P=0.42), oxygen saturation <90% (15% vs 11%; P=0.07), inadequate oral intake (50% vs 43%; P=0.13), and rates of relapse events requiring rehospitalization within both 24 hours (0.6% vs 0.6%; P=0.88) and 7 days (1% vs 1%; P=0.90) of discharge.
DISCUSSION
In this large, multicenter, multiyear study of children hospitalized with bronchiolitis, we found that children present to a hospital in a relatively narrow time frame, but their time to recovery in the hospital is highly variable. Nonetheless, 96% of children continued to improve once they had: (1) improving or stable retractions rated as none/mild, (2) a decreasing or stable RR by age, (3) estimated average RAO2 saturation 90% and lowest RAO2 saturation of 88%, and (4) were hydrated. The 4% of children who worsened after clinically improving were more likely to be age <2 months, born <37 weeks, and present with more severe distress (ie, severe retractions, inadequate oral intake, or apnea). Based on the low risk of worsening after clinical improvement, especially among children admitted to the regular ward (2%), we believe these 4 clinical criteria could be used as discharge criteria for this common pediatric illness with a predominantly monophasic clinical course.
Variability in hospital LOS for children with bronchiolitis exists in the United States[3] and internationally.[4, 5] Cheung and colleagues analyzed administrative data from over 75,000 children admitted for bronchiolitis in England between April 2007 and March 2010 and found sixfold variation in LOS between sites. They concluded that this LOS variability was due in part to providers' clinical decision making.[5] Srivastava and colleagues[23] addressed variable clinician decision making in bronchiolitis and 10 other common pediatric conditions by embedding discharge criteria developed by expert consensus into admission order sets. They found that for children with bronchiolitis, the embedded discharge criteria reduced the median LOS from 1.91 to 1.87 days. In contrast to the single‐center data presented by White and colleagues,[24] the prospective, multicenter MARC‐30 data provide a clear understanding of the normal clinical course for children hospitalized with bronchiolitis, determine if children clinically worsen after clinical improvement, and provide data about discharge criteria for children hospitalized with bronchiolitis. Although there is a lack of rigorous published data, the lower tract symptoms of bronchiolitis (eg, cough, retractions) are said to peak on days 5 to 7 of illness and then gradually resolve.[25] In the present study, we found that the time from the onset of difficulty breathing until hospital admission is less variable than the time from the onset of difficulty breathing until either clinical improvement or discharge. Although 75% of children have clinically improved within 7.5 days of difficulty breathing based on the IQR results, the remaining 25% may have a more prolonged recovery in the hospital of up to 3 weeks. Interestingly, prolonged recovery times from bronchiolitis have also been noted in children presenting to the ED[26] and in an outpatient population.[27] It is unclear why 20% to 25% of children at different levels of severity of illness have prolonged recovery from bronchiolitis, but this group of children requires further investigation.
Given the variability of recovery times, clinicians may have difficulty knowing when a child is ready for hospital discharge. One of the main stumbling blocks for discharge readiness in children with bronchiolitis is the interpretation of the oxygen saturation value.[6, 8, 9, 20, 28] However, it should be considered that interpreting the oxygen saturation in a child who is clinically improving in the hospital setting is different than interpreting the oxygen saturation of a child in the ED or the clinic whose clinical course is less certain.[22] In the hospital setting, using the oxygen saturation value in in the AAP guideline,[9] 4% of children clinically worsened after they met the improvement criteria, a clinical pattern observed previously with supplemental oxygen.[28] This unpredictability may explain some of the variation in providers' clinical decision making.[5] The children who worsened, and therefore deserve more cautious discharge planning, were young (<2 months), premature (<37 weeks gestational age), and presented in more severe distress. Those children admitted to the ICU from the ED worsened more commonly than children admitted to the ward (19% vs 2%). Interestingly, the viral etiology of the child's bronchiolitis did not influence whether a child worsened after reaching the improvement criteria. Therefore, although children with RV bronchiolitis have a shorter hospital LOS than children with RSV bronchiolitis,[11] the pattern of recovery did not differ by viral etiology.
In addition to unsafe discharges, clinicians may be concerned about the possibility of readmissions. Although somewhat controversial, hospital readmission is being used as a quality of care metric.[29, 30, 31] One response to minimize readmissions would be for clinicians to observe children for longer than clinically indicated.[32] However, shorter LOS is not necessarily associated with increased readmission rates.[33] Given that the geometric mean of hospital charges per child with bronchiolitis increased from $6380 in 2000 to $8530 in 2009,[34] the potential for safely reducing hospital LOS by using the discharge criteria proposed in the current study instead of other criteria[8] may net substantial cost savings. Furthermore, reducing LOS would decrease the time children expose others to these respiratory viruses and possibly reduce medical errors.[35]
Our study has some potential limitations. Because the study participants were all hospitalized, these data do not inform admission or discharge decisions from either the ED or the clinic; but other data address those clinical scenarios.[22] Also, the 16 sites that participated in this study were large, urban teaching hospitals. Consequently, these results are not necessarily generalizable to smaller community hospitals. Although numerous data points were required to enter the analytic cohort, only 9% of the sample was excluded for missing data. There were 214 children who did not meet our improvement criteria by the time of discharge. Although the inability to include these children in the analysis may be seen as a limitation, this practice variability underscores the need for more data about discharging hospitalized children with bronchiolitis. Last, site teams reviewed medical records daily. More frequent recording of the clinical course would have yielded more granular data, but the current methodology replicates how data are generally presented during patient care rounds, when decisions about suitability for discharge are often considered.
CONCLUSION
We documented in this large multicenter study that most children hospitalized with bronchiolitis had a wide range of time to recovery, but the vast majority continued to improve once they reached the identified clinical criteria that predict a safe discharge to home. The children who worsened after clinical improvement were more likely to be younger, premature infants presenting in more severe distress. Although additional prospective validation of these hospital discharge criteria is warranted, these data may help clinicians make more evidence‐based discharge decisions for a common pediatric illness with high practice variation, both in the United States[3] and in other countries.[4, 5]
Acknowledgements
Collaborators in the MARC‐30 Study: Besh Barcega, MD, Loma Linda University Children's Hospital, Loma Linda, CA; John Cheng, MD, Children's Healthcare of Atlanta at Egleston, Atlanta, GA; Dorothy Damore, MD, New York Presbyterian Hospital‐Cornell, New York, NY; Carlos Delgado, MD, Children's Healthcare of Atlanta at Egleston, Atlanta, GA; Haitham Haddad, MD, Rainbow Babies & Children's Hospital, Cleveland, OH; Paul Hain, MD, Monroe Carell Jr. Children's Hospital at Vanderbilt, Nashville, TN; Frank LoVecchio, DO, Maricopa Medical Center, Phoenix, AZ; Charles Macias, MD MPH, Texas Children's Hospital, Houston, TX; Jonathan Mansbach, MD, MPH, Boston Children's Hospital, Boston, MA; Eugene Mowad, MD, Akron Children's Hospital, Akron, OH; Brian Pate, MD, Children's Mercy Hospital, Kansas City, MO; Mark Riederer, MD, Monroe Carell Jr. Children's Hospital at Vanderbilt, Nashville, TN; M. Jason Sanders, MD, Children's Memorial Hermann Hospital, Houston, TX; Alan R. Schroeder, MD, Santa Clara Valley Medical Center, San Jose, CA; Nikhil Shah, MD, New York Presbyterian Hospital‐Cornell, New York, NY; Michelle Stevenson, MD, MS, Kosair Children's Hospital, Louisville, KY; Erin Stucky Fisher, MD, Rady Children's Hospital, San Diego, CA; Stephen Teach, MD, MPH, Children's National Medical Center, Washington, DC; Lisa Zaoutis, MD, Children's Hospital of Philadelphia, Philadelphia, PA.
Disclosures: This study was supported by grants U01 AI‐67693 and K23 AI‐77801 from the National Institutes of Health (Bethesda, MD). The content of this manuscript is solely the responsibility of the authors and does not necessarily represent the official views of the National Institute of Allergy and Infectious Diseases or the National Institutes of Health. Drs. Mansbach and Piedra have provided consultation to Regeneron Pharmaceuticals. Otherwise, no authors report any potential conflicts of interest, including relevant financial interests, activities, relationships, and affiliations.
- Infectious disease hospitalizations among infants in the United States. Pediatrics. 2008;121(2):244–252. , , , , .
- “A hospital is no place to be sick” Samuel Goldwyn (1882–1974). Arch Dis Child. 2009;94(8):565–566. .
- Variation in inpatient diagnostic testing and management of bronchiolitis. Pediatrics. 2005;115(4):878–884. , , , , ,
- International variation in the management of infants hospitalized with respiratory syncytial virus. International RSV Study Group. Eur J Pediatr. 1998;157(3):215–220. , , ,
- Population variation in admission rates and duration of inpatient stay for bronchiolitis in England. Arch Dis Child. 2013;98(1):57–59. , , , , .
- Impact of pulse oximetry and oxygen therapy on length of stay in bronchiolitis hospitalizations. Arch Pediatr Adolesc Med. 2004;158(6):527–530. , , , .
- Pulse oximetry in pediatric practice. Pediatrics. 2011;128(4):740–752. , , .
- Scottish Intercollegiate Guidelines Network. Bronchiolitis in children (SIGN 91). In: NHS Quality Improvement Scotland. Edinburgh, Scotland: Scottish Intercollegiate Guidelines Network; 2006.
- Diagnosis and management of bronchiolitis. Pediatrics. 2006;118(4):1774–1793. , , , et al.
- Prospective multicenter study of children with bronchiolitis requiring mechanical ventilation. Pediatrics. 2012;130(3):e492–e500. , , , et al.
- Prospective multicenter study of viral etiology and hospital length of stay in children with severe bronchiolitis. Arch Pediatr Adolesc Med. 2012;166(8):700–706. , , , et al.
- Apnea in children hospitalized with bronchiolitis. Pediatrics. 2013;132(5):e1194–e1201. , , , et al.
- Evaluation of the cardiovascular system: history and physical evaluation. In: Kliegman RM, Stanton BF, St. Geme JW III, Schor NF, Behrman RF, eds. Nelson Textbook of Pediatrics. Philadelphia, PA: Elsevier Saunders; 2011:1529–1536. .
- Apnea in children hospitalized with bronchiolitis. Pediatrics. 2013;132(5):e1194–e1201. , , , et al.
- Respiratory viral infections in patients with chronic, obstructive pulmonary disease. J Infect. 2005;50(4):322–330. , , , et al.
- Evaluation of real‐time PCR for diagnosis of Bordetella pertussis infection. BMC Infect Dis. 2006;6:62. , , , .
- Evaluation of three real‐time PCR assays for detection of Mycoplasma pneumoniae in an outbreak investigation. J Clin Microbiol. 2008;46(9):3116–3118. , , , , .
- Normal ranges of heart rate and respiratory rate in children from birth to 18 years of age: a systematic review of observational studies. Lancet. 2011;377(9770):1011–1018. , , , et al.
- Development of heart and respiratory rate percentile curves for hospitalized children. Pediatrics. 2013;131(4):e1150–e1157. , , , , , .
- Effect of oxygen supplementation on length of stay for infants hospitalized with acute viral bronchiolitis. Pediatrics. 2008;121(3):470–475. , .
- Longitudinal assessment of hemoglobin oxygen saturation in healthy infants during the first 6 months of age. Collaborative Home Infant Monitoring Evaluation (CHIME) Study Group. J Pediatr. 1999;135(5):580–586. , , , et al.
- Prospective multicenter study of bronchiolitis: predicting safe discharges from the emergency department. Pediatrics. 2008;121(4):680–688. , , , et al.
- Delays in discharge in a tertiary care pediatric hospital. J Hosp Med. 2009;4(8):481–485. , , , et al.
- Using quality improvement to optimise paediatric discharge efficiency. BMJ Qual Saf. 2014;23(5):428–436. , , , et al.
- Bronchiolitis in infants and children: treatment; outcome; and prevention. In: Torchia M, ed. UpToDate. Alphen aan den Rijn, the Netherlands; Wolters Kluwer Health; 2013. , .
- Duration of illness in infants with bronchiolitis evaluated in the emergency department. Pediatrics. 2010;126(2):285–290. , .
- Duration of illness in ambulatory children diagnosed with bronchiolitis. Arch Pediatr Adolesc Med. 2000;154(10):997–1000. , , .
- A clinical pathway for bronchiolitis is effective in reducing readmission rates. J Pediatr. 2005;147(5):622–626. , , , et al.
- Measuring hospital quality using pediatric readmission and revisit rates. Pediatrics. 2013;132(3):429–436. , , , et al.
- Pediatric readmission prevalence and variability across hospitals. JAMA. 2013;309(4):372–380. , , , et al.
- Preventability of early readmissions at a children's hospital. Pediatrics. 2013;131(1):e171–e181. , , , , , .
- Hospital readmission: quality indicator or statistical inevitability? Pediatrics. 2013;132(3):569–570. , .
- Children's hospitals with shorter lengths of stay do not have higher readmission rates. J Pediatr. 2013;163(4):1034–1038.e1. , , , et al.
- Trends in bronchiolitis hospitalizations in the United States, 2000–2009. Pediatrics. 2013;132(1):28–36. , , , , .
- Preventable adverse events in infants hospitalized with bronchiolitis. Pediatrics. 2005;116(3):603–608. , , , .
Although bronchiolitis is the leading cause of hospitalization for US infants,[1] there is a lack of basic prospective data about the expected inpatient clinical course and ongoing uncertainty about when a hospitalized child is ready for discharge to home.[2] This lack of data about children's readiness for discharge may result in variable hospital length‐of‐stay (LOS).[3, 4, 5]
One specific source of variability in discharge readiness and LOS variability may be the lack of consensus about safe threshold oxygen saturation values for discharge in children hospitalized with bronchiolitis.[6, 7] In 2006, the Scottish Intercollegiate Guidelines Network recommended a discharge room air oxygen (RAO2) saturation threshold of 95%.[8] The same year, the American Academy of Pediatrics (AAP) bronchiolitis clinical practice guideline stated that oxygen is not needed for children with RAO2 saturations 90% who are feeding well and have minimal respiratory distress.[9] There is a need for prospective studies to help clinicians make evidenced‐based discharge decisions for this common condition.
We performed a prospective, multicenter, multiyear study[10, 11, 12] to examine the typical inpatient clinical course of and to develop hospital discharge guidelines for children age <2 years hospitalized with bronchiolitis. We hypothesized that children would not worsen clinically and would be safe to discharge home once their respiratory status improved and they were able to remain hydrated.
METHODS
Study Design and Population
We conducted a prospective, multicenter cohort study for 3 consecutive years during the 2007 to 2010 winter seasons, as part of the Multicenter Airway Research Collaboration (MARC), a program of the Emergency Medicine Network (
All patients were treated at the discretion of the treating physician. Inclusion criteria were an attending physician's diagnosis of bronchiolitis, age <2 years, and the ability of the parent/guardian to give informed consent. The exclusion criteria were previous enrollment and transfer to a participating hospital >48 hours after the original admission time. Therefore, children with comorbid conditions were included in this study. All consent and data forms were translated into Spanish. The institutional review board at each of the 16 participating hospitals approved the study.
Of the 2207 enrolled children, we excluded 109 (5%) children with a hospital LOS <1 day due to inadequate time to capture the required data for the present analysis. Among the 2098 remaining children, 1916 (91%) had daily inpatient data on all factors used to define clinical improvement and clinical worsening. Thus, the analytic cohort was comprised of 1916 children hospitalized for bronchiolitis.
Data Collection
Investigators conducted detailed structured interviews. Chart reviews were conducted to obtain preadmission and daily hospital clinical data including respiratory rates, daily respiratory rate trends, degree of retractions, oxygen saturation, daily oxygen saturation trends, medical management, and disposition. These data were manually reviewed, and site investigators were queried about missing data and discrepancies. A follow‐up telephone interview was conducted with families 1 week after discharge to examine relapse events at both 24 hours and 7 days.
We used the question: How long ago did the following symptoms [eg, difficulty breathing] begin [for the] current illness? to estimate the onset of the current illness. Pulse was categorized as low, normal, or high based on age‐related heart rate values.[13] Presence of apnea was recorded daily by site investigators.[14]
Nasopharyngeal Aspirate Collection and Virology Testing
As described previously, site teams used a standardized protocol to collect nasopharyngeal aspirates,[11] which were tested for respiratory syncytial virus (RSV) types A and B; rhinovirus (RV); parainfluenza virus types 1, 2, and 3; influenza virus types A and B; 2009 novel H1N1; human metapneumovirus; coronaviruses NL‐63, HKU1, OC43, and 229E; enterovirus, and adenovirus using polymerase chain reaction.[11, 15, 16, 17]
Defining Clinical Improvement and Worsening
Clinical improvement criteria were based on the 2006 AAP guidelines.[9] For respiratory rate and oxygen saturation, clinicians estimated average daily respiratory rate and oxygen saturation based on the recorded readings from the previous 24 hours. This estimation reflects the process clinicians use when rounding on their hospitalized patients, and thus may be more similar to standard clinical practice than a calculated mean. The respiratory rate criteria are adjusted for age.[18, 19] For daily estimated average oxygen saturation we used the AAP criteria of RAO2 saturation of 90%. Considering that oxygen saturation is the main determinant of LOS,[20] healthy infants age <6 months may have transient oxygen saturations of around 80%,[21] and that errors in estimation may occur, we included a lowest RAO2 of 88% in our improvement criteria. By combining the dichotomized estimated oxygen saturation (90% or not) with the lower limit of 88%, there was little room for erroneous conclusions. A child was considered clinically improved on the earliest date he/she met all of the following criteria: (1) none or mild retractions and improved or stable retractions compared with the previous inpatient day; (2) daily estimated average respiratory rate (RR) <60 breaths per minute for age <6 months, <55 breaths/minute for age 6 to 11 months, and <45 breaths/minute for age 12 months with a decreasing or stable trend over the course of the current day; (3) daily estimated average RAO2 saturation 90%, lowest RAO2 saturation 88%[21]; and (4) not receiving intravenous (IV) fluids or for children receiving IV fluids a clinician report of the child maintaining oral hydration. Children who reached the clinical improvement criteria were considered clinically worse if they required intensive care or had the inverse of 1 of the improvement criteria: moderate/severe retractions that were worse compared with the previous inpatient day, daily average RR 60 with an increasing trend over the current day, need for oxygen, or need for IV fluids.
Statistical Analyses
All analyses were performed using Stata 12.0 (StataCorp, College Station, TX). Data are presented as proportions with 95% confidence intervals (95% CIs), means with standard deviations, and medians with interquartile ranges (IQR). To examine potential factors associated with clinical worsening after reaching clinical improvement, we used 2, Fisher exact, Student t test, and Kruskall‐Wallis tests, as appropriate.
Adjusted analyses used generalized linear mixed models with a logit link to identify independent risk factors for worsening after reaching clinical improvement. Fixed effects for patient‐level factors and a random site effect were used. Factors were tested for inclusion in the multivariable model if they were found to be associated with worsening in unadjusted analyses (P<0.20) or were considered clinically important. Results are reported as odds ratios with 95% CIs.
We performed several sensitivity analyses to evaluate these improvement criteria: (1) we excluded the lowest RAO2 saturation requirement of 88%, (2) we examined a 94% daily estimated average RAO2 saturation threshold,[22] (3) we examined a 95% daily estimated average RAO2 saturation threshold,[8] and (4) we examined children age <12 months with no history of wheeze.
RESULTS
There were 1916 children hospitalized with bronchiolitis with data on all factors used to define clinical improvement and clinical worsening. The median number of days from the beginning of difficulty breathing until admission was 2 days (IQR, 15.5 days; range, 18 days) and from the beginning of difficulty breathing until clinical improvement was 4 days (IQR, 37.5 days; range, 133 days) (Figure 1). The variance for days to admission was significantly less than the variance for days to clinical improvement (P<0.001).
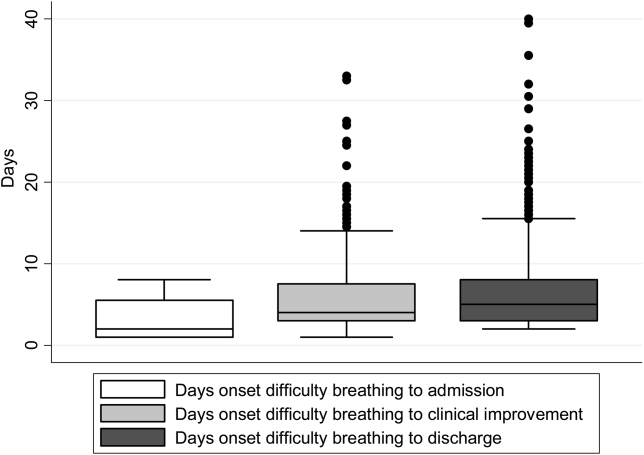
In this observational study, clinicians discharged 214 (11%) of the 1916 children before meeting the definition of clinical improvement. Thus, 1702 (89%; 95% CI: 87%‐90%) children reached the clinical improvement criteria, had a LOS >1 day, and had data on all factors (Figure 2).
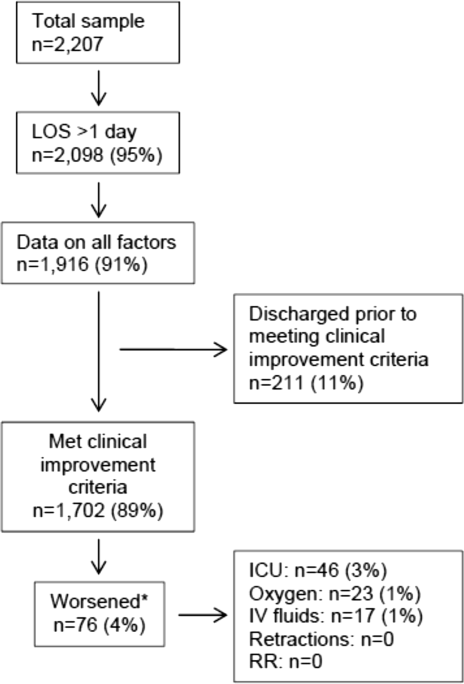
Of the 1702 children who met the clinical improvement criteria, there were 76 children (4%; 95% CI: 3%5%) who worsened (Figure 2). The worsening occurred within a median of 1 day (IQR, 13 days) of clinical improvement. Forty‐six (3%) of the children required transfer to the ICU (1 required intubation, 1 required continuous positive airway pressure, and 4 had apnea), 23 (1%) required oxygen, and 17 (1%) required IV fluids. Eight percent of children met multiple criteria for worsening. A comparison between children who did and did not worsen is shown in Table 1. In general, children who worsened after improvement were younger and born earlier. These children also presented in more severe respiratory distress, had moderate or severe retractions, oxygen saturation <85% at hospitalization, inadequate oral intake, and apnea documented during the hospitalization. Neither viral etiology nor site of care influenced whether the children worsened after improving. However, stratified analysis of children based on initial location of admission (ie, ICU or ward) showed that among the children admitted to the ICU from the emergency department (ED), 89% met the improvement criteria and 19% clinically worsened. In contrast, among children admitted to the ward from the ED, 89% met the improvement criteria, and only 2% clinically worsened. Stratified multivariable models based on the initial location of admission from the ED (ie, ICU or ward) were not possible due to small sample sizes after stratification. None of these children had relapse events requiring rehospitalization within either 24 hours or 7 days of discharge.
Did Not Worsen, n=1,626 | Worsened, n=76 | P Value | |
---|---|---|---|
| |||
Demographic characteristics | |||
Age <2 months, % | 29 | 57 | <0.001 |
Month of birth, % | 0.02 | ||
OctoberMarch | 61 | 75 | |
AprilSeptember | 39 | 25 | |
Sex, % | 0.51 | ||
Male | 59 | 55 | |
Female | 41 | 45 | |
Race, % | 0.050 | ||
White | 63 | 58 | |
Black | 23 | 34 | |
Other or missing | 14 | 8 | |
Hispanic ethnicity, % | 37 | 22 | 0.01 |
Insurance, % | 0.87 | ||
Nonprivate | 68 | 67 | |
Private | 32 | 33 | |
Medical history | |||
Gestational age <37 weeks, % | 23 | 39 | 0.002 |
Birth weight, % | 0.52 | ||
<5 lbs | 13 | 12 | |
5 lbs | 34 | 41 | |
7 lbs | 53 | 47 | |
Mother's age, median (IQR) | 27 (2333) | 27 (2233) | 0.54 |
Is or was breastfed, % | 61 | 51 | 0.10 |
Smoked during pregnancy, % | 15 | 20 | 0.22 |
Exposure to smoke, % | 13 | 20 | 0.11 |
Family history of asthma, % | 0.89 | ||
Neither parent | 68 | 64 | |
Either mother or father | 27 | 30 | |
Both parents | 4 | 4 | |
Do not know/missing | 2 | 1 | |
History of wheezing, % | 23 | 17 | 0.24 |
History of eczema, % | 16 | 7 | 0.04 |
History of intubation, % | 9 | 12 | 0.50 |
Major, relevant, comorbid medical disorder, % | 20 | 24 | 0.46 |
Current illness | |||
When difficulty breathing began, preadmission, % | 0.63 | ||
1 day | 70 | 75 | |
<1 day | 28 | 23 | |
No difficulty preadmission | 2 | 3 | |
Weight, lbs, median (IQR) | 12.3 (8.817.4) | 9.0 (6.613.2) | 0.001 |
Temperature, F, median (IQR) | 99.5 (98.6100.6) | 99.4 (98.1100.4) | 0.06 |
Pulse, beats per minute by age | 0.82 | ||
Low | 0.3 | 0 | |
Normal | 48 | 46 | |
High | 51 | 54 | |
Respiratory rate, breaths per minute, median (IQR) | 48 (4060) | 48 (3864) | 0.28 |
Retractions, % | 0.001 | ||
None | 22 | 25 | |
Mild | 43 | 24 | |
Moderate | 26 | 33 | |
Severe | 4 | 12 | |
Missing | 5 | 7 | |
Oxygen saturation by pulse oximetry or ABG, % | 0.001 | ||
<85 | 4 | 12 | |
8587.9 | 3 | 4 | |
8889.9 | 5 | 0 | |
9093.9 | 18 | 11 | |
94 | 72 | 73 | |
Oral intake, % | <0.001 | ||
Adequate | 45 | 22 | |
Inadequate | 42 | 63 | |
Missing | 13 | 14 | |
Presence of apnea, % | 7 | 24 | <0.001 |
RSV‐A, % | 44 | 41 | 0.54 |
RSV‐B, % | 30 | 25 | 0.36 |
HRV, % | 24 | 24 | 0.88 |
Chest x‐ray results during ED/preadmission visit | |||
Atelectasis | 12 | 13 | 0.77 |
Infiltrate | 13 | 11 | 0.50 |
Hyperinflated | 18 | 21 | 0.47 |
Peribronchial cuffing/thickening | 23 | 17 | 0.32 |
Normal | 14 | 16 | 0.75 |
White blood count, median (IQR) | 11.2 (8.714.4) | 11.9 (9.214.4) | 0.60 |
Platelet count, median (IQR) | 395 (317490) | 430 (299537) | 0.56 |
Sodium, median (IQR) | 138 (136140) | 137 (135138) | 0.19 |
Hospital length of stay, median (IQR) | 2 (14) | 4.5 (28) | <0.001 |
One‐week follow‐up | |||
Relapse within 24 hours of hospital discharge requiring hospital admission, % | 0.5 | 0 | 0.56 |
Relapse within 7 days of hospital discharge requiring hospital admission, % | 1 | 0 | 0.35 |
On multivariable analysis (Table 2), independent risk factors for worsening after reaching the clinical improvement criteria were young age, preterm birth, and presenting to care with more severe bronchiolitis represented by severe retractions, inadequate oral intake, or apnea. To further evaluate the improvement criteria in the current analysis, multiple sensitivity analyses were conducted. The frequency of clinical worsening after reaching the improvement criteria was stable when we examined different RA02 criteria in sensitivity analyses: (1) excluding RA02 as a criterion for improvement: 90% met improvement criteria and 4% experienced clinical worsening, (2) changing the average RA02 threshold for clinical improvement to 94%: 62% met improvement criteria and 6% experienced clinical worsening, and (3) changing the average RA02 threshold for clinical improvement to 95%: 47% met improvement criteria and 5% experienced clinical worsening. Furthermore, stratifying by age <2 months and restricting to more stringent definitions of bronchiolitis (ie, age <1 year or age <1 year+no history of wheezing) also did not materially change the results (see Supporting Figure 1 in the online version of this article).
Odds Ratio | 95% CI | P Value | |
---|---|---|---|
| |||
Age <2 months | 3.51 | 2.07‐5.94 | <0.001 |
Gestational age <37 weeks | 1.94 | 1.13‐3.32 | 0.02 |
Retractions | |||
None | 1.30 | 0.80‐3.23 | 0.19 |
Mild | 1.0 | Reference | |
Moderate | 1.91 | 0.99‐3.71 | 0.06 |
Severe | 5.55 | 2.1214.50 | <0.001 |
Missing | 1.70 | 0.53‐5.42 | 0.37 |
Oral intake | |||
Adequate | 1.00 | Reference | |
Inadequate | 2.54 | 1.39‐4.62 | 0.002 |
Unknown/missing | 1.88 | 0.79‐4.44 | 0.15 |
Presence of apnea | 2.87 | 1.45‐5.68 | 0.003 |
We compared the 214 children who were discharged prior to reaching clinical improvement with the 1702 children who reached the clinical improvement criteria. The 214 children were less likely to be age <2 months (22% vs 30%; P=0.02). These 2 groups (214 vs 1702) were similar with respect to severe retractions (2% vs 4%; P=0.13), median respiratory rate (48 vs 48; P=0.42), oxygen saturation <90% (15% vs 11%; P=0.07), inadequate oral intake (50% vs 43%; P=0.13), and rates of relapse events requiring rehospitalization within both 24 hours (0.6% vs 0.6%; P=0.88) and 7 days (1% vs 1%; P=0.90) of discharge.
DISCUSSION
In this large, multicenter, multiyear study of children hospitalized with bronchiolitis, we found that children present to a hospital in a relatively narrow time frame, but their time to recovery in the hospital is highly variable. Nonetheless, 96% of children continued to improve once they had: (1) improving or stable retractions rated as none/mild, (2) a decreasing or stable RR by age, (3) estimated average RAO2 saturation 90% and lowest RAO2 saturation of 88%, and (4) were hydrated. The 4% of children who worsened after clinically improving were more likely to be age <2 months, born <37 weeks, and present with more severe distress (ie, severe retractions, inadequate oral intake, or apnea). Based on the low risk of worsening after clinical improvement, especially among children admitted to the regular ward (2%), we believe these 4 clinical criteria could be used as discharge criteria for this common pediatric illness with a predominantly monophasic clinical course.
Variability in hospital LOS for children with bronchiolitis exists in the United States[3] and internationally.[4, 5] Cheung and colleagues analyzed administrative data from over 75,000 children admitted for bronchiolitis in England between April 2007 and March 2010 and found sixfold variation in LOS between sites. They concluded that this LOS variability was due in part to providers' clinical decision making.[5] Srivastava and colleagues[23] addressed variable clinician decision making in bronchiolitis and 10 other common pediatric conditions by embedding discharge criteria developed by expert consensus into admission order sets. They found that for children with bronchiolitis, the embedded discharge criteria reduced the median LOS from 1.91 to 1.87 days. In contrast to the single‐center data presented by White and colleagues,[24] the prospective, multicenter MARC‐30 data provide a clear understanding of the normal clinical course for children hospitalized with bronchiolitis, determine if children clinically worsen after clinical improvement, and provide data about discharge criteria for children hospitalized with bronchiolitis. Although there is a lack of rigorous published data, the lower tract symptoms of bronchiolitis (eg, cough, retractions) are said to peak on days 5 to 7 of illness and then gradually resolve.[25] In the present study, we found that the time from the onset of difficulty breathing until hospital admission is less variable than the time from the onset of difficulty breathing until either clinical improvement or discharge. Although 75% of children have clinically improved within 7.5 days of difficulty breathing based on the IQR results, the remaining 25% may have a more prolonged recovery in the hospital of up to 3 weeks. Interestingly, prolonged recovery times from bronchiolitis have also been noted in children presenting to the ED[26] and in an outpatient population.[27] It is unclear why 20% to 25% of children at different levels of severity of illness have prolonged recovery from bronchiolitis, but this group of children requires further investigation.
Given the variability of recovery times, clinicians may have difficulty knowing when a child is ready for hospital discharge. One of the main stumbling blocks for discharge readiness in children with bronchiolitis is the interpretation of the oxygen saturation value.[6, 8, 9, 20, 28] However, it should be considered that interpreting the oxygen saturation in a child who is clinically improving in the hospital setting is different than interpreting the oxygen saturation of a child in the ED or the clinic whose clinical course is less certain.[22] In the hospital setting, using the oxygen saturation value in in the AAP guideline,[9] 4% of children clinically worsened after they met the improvement criteria, a clinical pattern observed previously with supplemental oxygen.[28] This unpredictability may explain some of the variation in providers' clinical decision making.[5] The children who worsened, and therefore deserve more cautious discharge planning, were young (<2 months), premature (<37 weeks gestational age), and presented in more severe distress. Those children admitted to the ICU from the ED worsened more commonly than children admitted to the ward (19% vs 2%). Interestingly, the viral etiology of the child's bronchiolitis did not influence whether a child worsened after reaching the improvement criteria. Therefore, although children with RV bronchiolitis have a shorter hospital LOS than children with RSV bronchiolitis,[11] the pattern of recovery did not differ by viral etiology.
In addition to unsafe discharges, clinicians may be concerned about the possibility of readmissions. Although somewhat controversial, hospital readmission is being used as a quality of care metric.[29, 30, 31] One response to minimize readmissions would be for clinicians to observe children for longer than clinically indicated.[32] However, shorter LOS is not necessarily associated with increased readmission rates.[33] Given that the geometric mean of hospital charges per child with bronchiolitis increased from $6380 in 2000 to $8530 in 2009,[34] the potential for safely reducing hospital LOS by using the discharge criteria proposed in the current study instead of other criteria[8] may net substantial cost savings. Furthermore, reducing LOS would decrease the time children expose others to these respiratory viruses and possibly reduce medical errors.[35]
Our study has some potential limitations. Because the study participants were all hospitalized, these data do not inform admission or discharge decisions from either the ED or the clinic; but other data address those clinical scenarios.[22] Also, the 16 sites that participated in this study were large, urban teaching hospitals. Consequently, these results are not necessarily generalizable to smaller community hospitals. Although numerous data points were required to enter the analytic cohort, only 9% of the sample was excluded for missing data. There were 214 children who did not meet our improvement criteria by the time of discharge. Although the inability to include these children in the analysis may be seen as a limitation, this practice variability underscores the need for more data about discharging hospitalized children with bronchiolitis. Last, site teams reviewed medical records daily. More frequent recording of the clinical course would have yielded more granular data, but the current methodology replicates how data are generally presented during patient care rounds, when decisions about suitability for discharge are often considered.
CONCLUSION
We documented in this large multicenter study that most children hospitalized with bronchiolitis had a wide range of time to recovery, but the vast majority continued to improve once they reached the identified clinical criteria that predict a safe discharge to home. The children who worsened after clinical improvement were more likely to be younger, premature infants presenting in more severe distress. Although additional prospective validation of these hospital discharge criteria is warranted, these data may help clinicians make more evidence‐based discharge decisions for a common pediatric illness with high practice variation, both in the United States[3] and in other countries.[4, 5]
Acknowledgements
Collaborators in the MARC‐30 Study: Besh Barcega, MD, Loma Linda University Children's Hospital, Loma Linda, CA; John Cheng, MD, Children's Healthcare of Atlanta at Egleston, Atlanta, GA; Dorothy Damore, MD, New York Presbyterian Hospital‐Cornell, New York, NY; Carlos Delgado, MD, Children's Healthcare of Atlanta at Egleston, Atlanta, GA; Haitham Haddad, MD, Rainbow Babies & Children's Hospital, Cleveland, OH; Paul Hain, MD, Monroe Carell Jr. Children's Hospital at Vanderbilt, Nashville, TN; Frank LoVecchio, DO, Maricopa Medical Center, Phoenix, AZ; Charles Macias, MD MPH, Texas Children's Hospital, Houston, TX; Jonathan Mansbach, MD, MPH, Boston Children's Hospital, Boston, MA; Eugene Mowad, MD, Akron Children's Hospital, Akron, OH; Brian Pate, MD, Children's Mercy Hospital, Kansas City, MO; Mark Riederer, MD, Monroe Carell Jr. Children's Hospital at Vanderbilt, Nashville, TN; M. Jason Sanders, MD, Children's Memorial Hermann Hospital, Houston, TX; Alan R. Schroeder, MD, Santa Clara Valley Medical Center, San Jose, CA; Nikhil Shah, MD, New York Presbyterian Hospital‐Cornell, New York, NY; Michelle Stevenson, MD, MS, Kosair Children's Hospital, Louisville, KY; Erin Stucky Fisher, MD, Rady Children's Hospital, San Diego, CA; Stephen Teach, MD, MPH, Children's National Medical Center, Washington, DC; Lisa Zaoutis, MD, Children's Hospital of Philadelphia, Philadelphia, PA.
Disclosures: This study was supported by grants U01 AI‐67693 and K23 AI‐77801 from the National Institutes of Health (Bethesda, MD). The content of this manuscript is solely the responsibility of the authors and does not necessarily represent the official views of the National Institute of Allergy and Infectious Diseases or the National Institutes of Health. Drs. Mansbach and Piedra have provided consultation to Regeneron Pharmaceuticals. Otherwise, no authors report any potential conflicts of interest, including relevant financial interests, activities, relationships, and affiliations.
Although bronchiolitis is the leading cause of hospitalization for US infants,[1] there is a lack of basic prospective data about the expected inpatient clinical course and ongoing uncertainty about when a hospitalized child is ready for discharge to home.[2] This lack of data about children's readiness for discharge may result in variable hospital length‐of‐stay (LOS).[3, 4, 5]
One specific source of variability in discharge readiness and LOS variability may be the lack of consensus about safe threshold oxygen saturation values for discharge in children hospitalized with bronchiolitis.[6, 7] In 2006, the Scottish Intercollegiate Guidelines Network recommended a discharge room air oxygen (RAO2) saturation threshold of 95%.[8] The same year, the American Academy of Pediatrics (AAP) bronchiolitis clinical practice guideline stated that oxygen is not needed for children with RAO2 saturations 90% who are feeding well and have minimal respiratory distress.[9] There is a need for prospective studies to help clinicians make evidenced‐based discharge decisions for this common condition.
We performed a prospective, multicenter, multiyear study[10, 11, 12] to examine the typical inpatient clinical course of and to develop hospital discharge guidelines for children age <2 years hospitalized with bronchiolitis. We hypothesized that children would not worsen clinically and would be safe to discharge home once their respiratory status improved and they were able to remain hydrated.
METHODS
Study Design and Population
We conducted a prospective, multicenter cohort study for 3 consecutive years during the 2007 to 2010 winter seasons, as part of the Multicenter Airway Research Collaboration (MARC), a program of the Emergency Medicine Network (
All patients were treated at the discretion of the treating physician. Inclusion criteria were an attending physician's diagnosis of bronchiolitis, age <2 years, and the ability of the parent/guardian to give informed consent. The exclusion criteria were previous enrollment and transfer to a participating hospital >48 hours after the original admission time. Therefore, children with comorbid conditions were included in this study. All consent and data forms were translated into Spanish. The institutional review board at each of the 16 participating hospitals approved the study.
Of the 2207 enrolled children, we excluded 109 (5%) children with a hospital LOS <1 day due to inadequate time to capture the required data for the present analysis. Among the 2098 remaining children, 1916 (91%) had daily inpatient data on all factors used to define clinical improvement and clinical worsening. Thus, the analytic cohort was comprised of 1916 children hospitalized for bronchiolitis.
Data Collection
Investigators conducted detailed structured interviews. Chart reviews were conducted to obtain preadmission and daily hospital clinical data including respiratory rates, daily respiratory rate trends, degree of retractions, oxygen saturation, daily oxygen saturation trends, medical management, and disposition. These data were manually reviewed, and site investigators were queried about missing data and discrepancies. A follow‐up telephone interview was conducted with families 1 week after discharge to examine relapse events at both 24 hours and 7 days.
We used the question: How long ago did the following symptoms [eg, difficulty breathing] begin [for the] current illness? to estimate the onset of the current illness. Pulse was categorized as low, normal, or high based on age‐related heart rate values.[13] Presence of apnea was recorded daily by site investigators.[14]
Nasopharyngeal Aspirate Collection and Virology Testing
As described previously, site teams used a standardized protocol to collect nasopharyngeal aspirates,[11] which were tested for respiratory syncytial virus (RSV) types A and B; rhinovirus (RV); parainfluenza virus types 1, 2, and 3; influenza virus types A and B; 2009 novel H1N1; human metapneumovirus; coronaviruses NL‐63, HKU1, OC43, and 229E; enterovirus, and adenovirus using polymerase chain reaction.[11, 15, 16, 17]
Defining Clinical Improvement and Worsening
Clinical improvement criteria were based on the 2006 AAP guidelines.[9] For respiratory rate and oxygen saturation, clinicians estimated average daily respiratory rate and oxygen saturation based on the recorded readings from the previous 24 hours. This estimation reflects the process clinicians use when rounding on their hospitalized patients, and thus may be more similar to standard clinical practice than a calculated mean. The respiratory rate criteria are adjusted for age.[18, 19] For daily estimated average oxygen saturation we used the AAP criteria of RAO2 saturation of 90%. Considering that oxygen saturation is the main determinant of LOS,[20] healthy infants age <6 months may have transient oxygen saturations of around 80%,[21] and that errors in estimation may occur, we included a lowest RAO2 of 88% in our improvement criteria. By combining the dichotomized estimated oxygen saturation (90% or not) with the lower limit of 88%, there was little room for erroneous conclusions. A child was considered clinically improved on the earliest date he/she met all of the following criteria: (1) none or mild retractions and improved or stable retractions compared with the previous inpatient day; (2) daily estimated average respiratory rate (RR) <60 breaths per minute for age <6 months, <55 breaths/minute for age 6 to 11 months, and <45 breaths/minute for age 12 months with a decreasing or stable trend over the course of the current day; (3) daily estimated average RAO2 saturation 90%, lowest RAO2 saturation 88%[21]; and (4) not receiving intravenous (IV) fluids or for children receiving IV fluids a clinician report of the child maintaining oral hydration. Children who reached the clinical improvement criteria were considered clinically worse if they required intensive care or had the inverse of 1 of the improvement criteria: moderate/severe retractions that were worse compared with the previous inpatient day, daily average RR 60 with an increasing trend over the current day, need for oxygen, or need for IV fluids.
Statistical Analyses
All analyses were performed using Stata 12.0 (StataCorp, College Station, TX). Data are presented as proportions with 95% confidence intervals (95% CIs), means with standard deviations, and medians with interquartile ranges (IQR). To examine potential factors associated with clinical worsening after reaching clinical improvement, we used 2, Fisher exact, Student t test, and Kruskall‐Wallis tests, as appropriate.
Adjusted analyses used generalized linear mixed models with a logit link to identify independent risk factors for worsening after reaching clinical improvement. Fixed effects for patient‐level factors and a random site effect were used. Factors were tested for inclusion in the multivariable model if they were found to be associated with worsening in unadjusted analyses (P<0.20) or were considered clinically important. Results are reported as odds ratios with 95% CIs.
We performed several sensitivity analyses to evaluate these improvement criteria: (1) we excluded the lowest RAO2 saturation requirement of 88%, (2) we examined a 94% daily estimated average RAO2 saturation threshold,[22] (3) we examined a 95% daily estimated average RAO2 saturation threshold,[8] and (4) we examined children age <12 months with no history of wheeze.
RESULTS
There were 1916 children hospitalized with bronchiolitis with data on all factors used to define clinical improvement and clinical worsening. The median number of days from the beginning of difficulty breathing until admission was 2 days (IQR, 15.5 days; range, 18 days) and from the beginning of difficulty breathing until clinical improvement was 4 days (IQR, 37.5 days; range, 133 days) (Figure 1). The variance for days to admission was significantly less than the variance for days to clinical improvement (P<0.001).
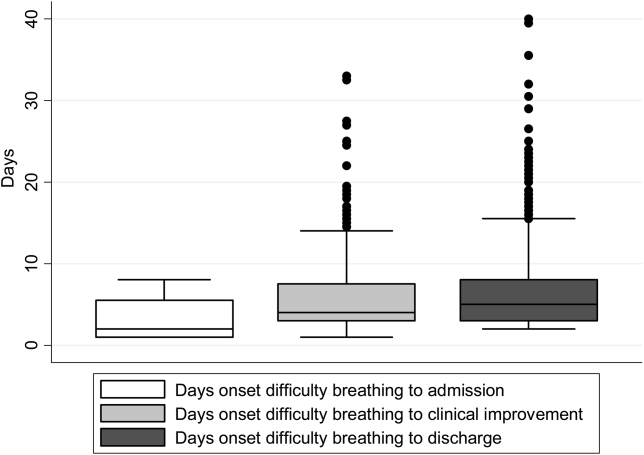
In this observational study, clinicians discharged 214 (11%) of the 1916 children before meeting the definition of clinical improvement. Thus, 1702 (89%; 95% CI: 87%‐90%) children reached the clinical improvement criteria, had a LOS >1 day, and had data on all factors (Figure 2).
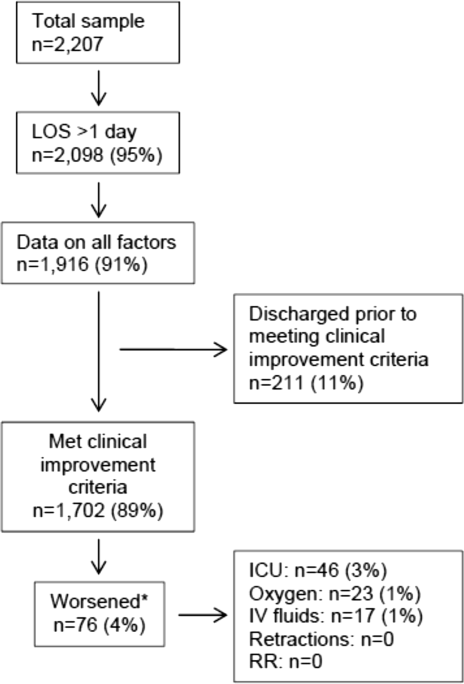
Of the 1702 children who met the clinical improvement criteria, there were 76 children (4%; 95% CI: 3%5%) who worsened (Figure 2). The worsening occurred within a median of 1 day (IQR, 13 days) of clinical improvement. Forty‐six (3%) of the children required transfer to the ICU (1 required intubation, 1 required continuous positive airway pressure, and 4 had apnea), 23 (1%) required oxygen, and 17 (1%) required IV fluids. Eight percent of children met multiple criteria for worsening. A comparison between children who did and did not worsen is shown in Table 1. In general, children who worsened after improvement were younger and born earlier. These children also presented in more severe respiratory distress, had moderate or severe retractions, oxygen saturation <85% at hospitalization, inadequate oral intake, and apnea documented during the hospitalization. Neither viral etiology nor site of care influenced whether the children worsened after improving. However, stratified analysis of children based on initial location of admission (ie, ICU or ward) showed that among the children admitted to the ICU from the emergency department (ED), 89% met the improvement criteria and 19% clinically worsened. In contrast, among children admitted to the ward from the ED, 89% met the improvement criteria, and only 2% clinically worsened. Stratified multivariable models based on the initial location of admission from the ED (ie, ICU or ward) were not possible due to small sample sizes after stratification. None of these children had relapse events requiring rehospitalization within either 24 hours or 7 days of discharge.
Did Not Worsen, n=1,626 | Worsened, n=76 | P Value | |
---|---|---|---|
| |||
Demographic characteristics | |||
Age <2 months, % | 29 | 57 | <0.001 |
Month of birth, % | 0.02 | ||
OctoberMarch | 61 | 75 | |
AprilSeptember | 39 | 25 | |
Sex, % | 0.51 | ||
Male | 59 | 55 | |
Female | 41 | 45 | |
Race, % | 0.050 | ||
White | 63 | 58 | |
Black | 23 | 34 | |
Other or missing | 14 | 8 | |
Hispanic ethnicity, % | 37 | 22 | 0.01 |
Insurance, % | 0.87 | ||
Nonprivate | 68 | 67 | |
Private | 32 | 33 | |
Medical history | |||
Gestational age <37 weeks, % | 23 | 39 | 0.002 |
Birth weight, % | 0.52 | ||
<5 lbs | 13 | 12 | |
5 lbs | 34 | 41 | |
7 lbs | 53 | 47 | |
Mother's age, median (IQR) | 27 (2333) | 27 (2233) | 0.54 |
Is or was breastfed, % | 61 | 51 | 0.10 |
Smoked during pregnancy, % | 15 | 20 | 0.22 |
Exposure to smoke, % | 13 | 20 | 0.11 |
Family history of asthma, % | 0.89 | ||
Neither parent | 68 | 64 | |
Either mother or father | 27 | 30 | |
Both parents | 4 | 4 | |
Do not know/missing | 2 | 1 | |
History of wheezing, % | 23 | 17 | 0.24 |
History of eczema, % | 16 | 7 | 0.04 |
History of intubation, % | 9 | 12 | 0.50 |
Major, relevant, comorbid medical disorder, % | 20 | 24 | 0.46 |
Current illness | |||
When difficulty breathing began, preadmission, % | 0.63 | ||
1 day | 70 | 75 | |
<1 day | 28 | 23 | |
No difficulty preadmission | 2 | 3 | |
Weight, lbs, median (IQR) | 12.3 (8.817.4) | 9.0 (6.613.2) | 0.001 |
Temperature, F, median (IQR) | 99.5 (98.6100.6) | 99.4 (98.1100.4) | 0.06 |
Pulse, beats per minute by age | 0.82 | ||
Low | 0.3 | 0 | |
Normal | 48 | 46 | |
High | 51 | 54 | |
Respiratory rate, breaths per minute, median (IQR) | 48 (4060) | 48 (3864) | 0.28 |
Retractions, % | 0.001 | ||
None | 22 | 25 | |
Mild | 43 | 24 | |
Moderate | 26 | 33 | |
Severe | 4 | 12 | |
Missing | 5 | 7 | |
Oxygen saturation by pulse oximetry or ABG, % | 0.001 | ||
<85 | 4 | 12 | |
8587.9 | 3 | 4 | |
8889.9 | 5 | 0 | |
9093.9 | 18 | 11 | |
94 | 72 | 73 | |
Oral intake, % | <0.001 | ||
Adequate | 45 | 22 | |
Inadequate | 42 | 63 | |
Missing | 13 | 14 | |
Presence of apnea, % | 7 | 24 | <0.001 |
RSV‐A, % | 44 | 41 | 0.54 |
RSV‐B, % | 30 | 25 | 0.36 |
HRV, % | 24 | 24 | 0.88 |
Chest x‐ray results during ED/preadmission visit | |||
Atelectasis | 12 | 13 | 0.77 |
Infiltrate | 13 | 11 | 0.50 |
Hyperinflated | 18 | 21 | 0.47 |
Peribronchial cuffing/thickening | 23 | 17 | 0.32 |
Normal | 14 | 16 | 0.75 |
White blood count, median (IQR) | 11.2 (8.714.4) | 11.9 (9.214.4) | 0.60 |
Platelet count, median (IQR) | 395 (317490) | 430 (299537) | 0.56 |
Sodium, median (IQR) | 138 (136140) | 137 (135138) | 0.19 |
Hospital length of stay, median (IQR) | 2 (14) | 4.5 (28) | <0.001 |
One‐week follow‐up | |||
Relapse within 24 hours of hospital discharge requiring hospital admission, % | 0.5 | 0 | 0.56 |
Relapse within 7 days of hospital discharge requiring hospital admission, % | 1 | 0 | 0.35 |
On multivariable analysis (Table 2), independent risk factors for worsening after reaching the clinical improvement criteria were young age, preterm birth, and presenting to care with more severe bronchiolitis represented by severe retractions, inadequate oral intake, or apnea. To further evaluate the improvement criteria in the current analysis, multiple sensitivity analyses were conducted. The frequency of clinical worsening after reaching the improvement criteria was stable when we examined different RA02 criteria in sensitivity analyses: (1) excluding RA02 as a criterion for improvement: 90% met improvement criteria and 4% experienced clinical worsening, (2) changing the average RA02 threshold for clinical improvement to 94%: 62% met improvement criteria and 6% experienced clinical worsening, and (3) changing the average RA02 threshold for clinical improvement to 95%: 47% met improvement criteria and 5% experienced clinical worsening. Furthermore, stratifying by age <2 months and restricting to more stringent definitions of bronchiolitis (ie, age <1 year or age <1 year+no history of wheezing) also did not materially change the results (see Supporting Figure 1 in the online version of this article).
Odds Ratio | 95% CI | P Value | |
---|---|---|---|
| |||
Age <2 months | 3.51 | 2.07‐5.94 | <0.001 |
Gestational age <37 weeks | 1.94 | 1.13‐3.32 | 0.02 |
Retractions | |||
None | 1.30 | 0.80‐3.23 | 0.19 |
Mild | 1.0 | Reference | |
Moderate | 1.91 | 0.99‐3.71 | 0.06 |
Severe | 5.55 | 2.1214.50 | <0.001 |
Missing | 1.70 | 0.53‐5.42 | 0.37 |
Oral intake | |||
Adequate | 1.00 | Reference | |
Inadequate | 2.54 | 1.39‐4.62 | 0.002 |
Unknown/missing | 1.88 | 0.79‐4.44 | 0.15 |
Presence of apnea | 2.87 | 1.45‐5.68 | 0.003 |
We compared the 214 children who were discharged prior to reaching clinical improvement with the 1702 children who reached the clinical improvement criteria. The 214 children were less likely to be age <2 months (22% vs 30%; P=0.02). These 2 groups (214 vs 1702) were similar with respect to severe retractions (2% vs 4%; P=0.13), median respiratory rate (48 vs 48; P=0.42), oxygen saturation <90% (15% vs 11%; P=0.07), inadequate oral intake (50% vs 43%; P=0.13), and rates of relapse events requiring rehospitalization within both 24 hours (0.6% vs 0.6%; P=0.88) and 7 days (1% vs 1%; P=0.90) of discharge.
DISCUSSION
In this large, multicenter, multiyear study of children hospitalized with bronchiolitis, we found that children present to a hospital in a relatively narrow time frame, but their time to recovery in the hospital is highly variable. Nonetheless, 96% of children continued to improve once they had: (1) improving or stable retractions rated as none/mild, (2) a decreasing or stable RR by age, (3) estimated average RAO2 saturation 90% and lowest RAO2 saturation of 88%, and (4) were hydrated. The 4% of children who worsened after clinically improving were more likely to be age <2 months, born <37 weeks, and present with more severe distress (ie, severe retractions, inadequate oral intake, or apnea). Based on the low risk of worsening after clinical improvement, especially among children admitted to the regular ward (2%), we believe these 4 clinical criteria could be used as discharge criteria for this common pediatric illness with a predominantly monophasic clinical course.
Variability in hospital LOS for children with bronchiolitis exists in the United States[3] and internationally.[4, 5] Cheung and colleagues analyzed administrative data from over 75,000 children admitted for bronchiolitis in England between April 2007 and March 2010 and found sixfold variation in LOS between sites. They concluded that this LOS variability was due in part to providers' clinical decision making.[5] Srivastava and colleagues[23] addressed variable clinician decision making in bronchiolitis and 10 other common pediatric conditions by embedding discharge criteria developed by expert consensus into admission order sets. They found that for children with bronchiolitis, the embedded discharge criteria reduced the median LOS from 1.91 to 1.87 days. In contrast to the single‐center data presented by White and colleagues,[24] the prospective, multicenter MARC‐30 data provide a clear understanding of the normal clinical course for children hospitalized with bronchiolitis, determine if children clinically worsen after clinical improvement, and provide data about discharge criteria for children hospitalized with bronchiolitis. Although there is a lack of rigorous published data, the lower tract symptoms of bronchiolitis (eg, cough, retractions) are said to peak on days 5 to 7 of illness and then gradually resolve.[25] In the present study, we found that the time from the onset of difficulty breathing until hospital admission is less variable than the time from the onset of difficulty breathing until either clinical improvement or discharge. Although 75% of children have clinically improved within 7.5 days of difficulty breathing based on the IQR results, the remaining 25% may have a more prolonged recovery in the hospital of up to 3 weeks. Interestingly, prolonged recovery times from bronchiolitis have also been noted in children presenting to the ED[26] and in an outpatient population.[27] It is unclear why 20% to 25% of children at different levels of severity of illness have prolonged recovery from bronchiolitis, but this group of children requires further investigation.
Given the variability of recovery times, clinicians may have difficulty knowing when a child is ready for hospital discharge. One of the main stumbling blocks for discharge readiness in children with bronchiolitis is the interpretation of the oxygen saturation value.[6, 8, 9, 20, 28] However, it should be considered that interpreting the oxygen saturation in a child who is clinically improving in the hospital setting is different than interpreting the oxygen saturation of a child in the ED or the clinic whose clinical course is less certain.[22] In the hospital setting, using the oxygen saturation value in in the AAP guideline,[9] 4% of children clinically worsened after they met the improvement criteria, a clinical pattern observed previously with supplemental oxygen.[28] This unpredictability may explain some of the variation in providers' clinical decision making.[5] The children who worsened, and therefore deserve more cautious discharge planning, were young (<2 months), premature (<37 weeks gestational age), and presented in more severe distress. Those children admitted to the ICU from the ED worsened more commonly than children admitted to the ward (19% vs 2%). Interestingly, the viral etiology of the child's bronchiolitis did not influence whether a child worsened after reaching the improvement criteria. Therefore, although children with RV bronchiolitis have a shorter hospital LOS than children with RSV bronchiolitis,[11] the pattern of recovery did not differ by viral etiology.
In addition to unsafe discharges, clinicians may be concerned about the possibility of readmissions. Although somewhat controversial, hospital readmission is being used as a quality of care metric.[29, 30, 31] One response to minimize readmissions would be for clinicians to observe children for longer than clinically indicated.[32] However, shorter LOS is not necessarily associated with increased readmission rates.[33] Given that the geometric mean of hospital charges per child with bronchiolitis increased from $6380 in 2000 to $8530 in 2009,[34] the potential for safely reducing hospital LOS by using the discharge criteria proposed in the current study instead of other criteria[8] may net substantial cost savings. Furthermore, reducing LOS would decrease the time children expose others to these respiratory viruses and possibly reduce medical errors.[35]
Our study has some potential limitations. Because the study participants were all hospitalized, these data do not inform admission or discharge decisions from either the ED or the clinic; but other data address those clinical scenarios.[22] Also, the 16 sites that participated in this study were large, urban teaching hospitals. Consequently, these results are not necessarily generalizable to smaller community hospitals. Although numerous data points were required to enter the analytic cohort, only 9% of the sample was excluded for missing data. There were 214 children who did not meet our improvement criteria by the time of discharge. Although the inability to include these children in the analysis may be seen as a limitation, this practice variability underscores the need for more data about discharging hospitalized children with bronchiolitis. Last, site teams reviewed medical records daily. More frequent recording of the clinical course would have yielded more granular data, but the current methodology replicates how data are generally presented during patient care rounds, when decisions about suitability for discharge are often considered.
CONCLUSION
We documented in this large multicenter study that most children hospitalized with bronchiolitis had a wide range of time to recovery, but the vast majority continued to improve once they reached the identified clinical criteria that predict a safe discharge to home. The children who worsened after clinical improvement were more likely to be younger, premature infants presenting in more severe distress. Although additional prospective validation of these hospital discharge criteria is warranted, these data may help clinicians make more evidence‐based discharge decisions for a common pediatric illness with high practice variation, both in the United States[3] and in other countries.[4, 5]
Acknowledgements
Collaborators in the MARC‐30 Study: Besh Barcega, MD, Loma Linda University Children's Hospital, Loma Linda, CA; John Cheng, MD, Children's Healthcare of Atlanta at Egleston, Atlanta, GA; Dorothy Damore, MD, New York Presbyterian Hospital‐Cornell, New York, NY; Carlos Delgado, MD, Children's Healthcare of Atlanta at Egleston, Atlanta, GA; Haitham Haddad, MD, Rainbow Babies & Children's Hospital, Cleveland, OH; Paul Hain, MD, Monroe Carell Jr. Children's Hospital at Vanderbilt, Nashville, TN; Frank LoVecchio, DO, Maricopa Medical Center, Phoenix, AZ; Charles Macias, MD MPH, Texas Children's Hospital, Houston, TX; Jonathan Mansbach, MD, MPH, Boston Children's Hospital, Boston, MA; Eugene Mowad, MD, Akron Children's Hospital, Akron, OH; Brian Pate, MD, Children's Mercy Hospital, Kansas City, MO; Mark Riederer, MD, Monroe Carell Jr. Children's Hospital at Vanderbilt, Nashville, TN; M. Jason Sanders, MD, Children's Memorial Hermann Hospital, Houston, TX; Alan R. Schroeder, MD, Santa Clara Valley Medical Center, San Jose, CA; Nikhil Shah, MD, New York Presbyterian Hospital‐Cornell, New York, NY; Michelle Stevenson, MD, MS, Kosair Children's Hospital, Louisville, KY; Erin Stucky Fisher, MD, Rady Children's Hospital, San Diego, CA; Stephen Teach, MD, MPH, Children's National Medical Center, Washington, DC; Lisa Zaoutis, MD, Children's Hospital of Philadelphia, Philadelphia, PA.
Disclosures: This study was supported by grants U01 AI‐67693 and K23 AI‐77801 from the National Institutes of Health (Bethesda, MD). The content of this manuscript is solely the responsibility of the authors and does not necessarily represent the official views of the National Institute of Allergy and Infectious Diseases or the National Institutes of Health. Drs. Mansbach and Piedra have provided consultation to Regeneron Pharmaceuticals. Otherwise, no authors report any potential conflicts of interest, including relevant financial interests, activities, relationships, and affiliations.
- Infectious disease hospitalizations among infants in the United States. Pediatrics. 2008;121(2):244–252. , , , , .
- “A hospital is no place to be sick” Samuel Goldwyn (1882–1974). Arch Dis Child. 2009;94(8):565–566. .
- Variation in inpatient diagnostic testing and management of bronchiolitis. Pediatrics. 2005;115(4):878–884. , , , , ,
- International variation in the management of infants hospitalized with respiratory syncytial virus. International RSV Study Group. Eur J Pediatr. 1998;157(3):215–220. , , ,
- Population variation in admission rates and duration of inpatient stay for bronchiolitis in England. Arch Dis Child. 2013;98(1):57–59. , , , , .
- Impact of pulse oximetry and oxygen therapy on length of stay in bronchiolitis hospitalizations. Arch Pediatr Adolesc Med. 2004;158(6):527–530. , , , .
- Pulse oximetry in pediatric practice. Pediatrics. 2011;128(4):740–752. , , .
- Scottish Intercollegiate Guidelines Network. Bronchiolitis in children (SIGN 91). In: NHS Quality Improvement Scotland. Edinburgh, Scotland: Scottish Intercollegiate Guidelines Network; 2006.
- Diagnosis and management of bronchiolitis. Pediatrics. 2006;118(4):1774–1793. , , , et al.
- Prospective multicenter study of children with bronchiolitis requiring mechanical ventilation. Pediatrics. 2012;130(3):e492–e500. , , , et al.
- Prospective multicenter study of viral etiology and hospital length of stay in children with severe bronchiolitis. Arch Pediatr Adolesc Med. 2012;166(8):700–706. , , , et al.
- Apnea in children hospitalized with bronchiolitis. Pediatrics. 2013;132(5):e1194–e1201. , , , et al.
- Evaluation of the cardiovascular system: history and physical evaluation. In: Kliegman RM, Stanton BF, St. Geme JW III, Schor NF, Behrman RF, eds. Nelson Textbook of Pediatrics. Philadelphia, PA: Elsevier Saunders; 2011:1529–1536. .
- Apnea in children hospitalized with bronchiolitis. Pediatrics. 2013;132(5):e1194–e1201. , , , et al.
- Respiratory viral infections in patients with chronic, obstructive pulmonary disease. J Infect. 2005;50(4):322–330. , , , et al.
- Evaluation of real‐time PCR for diagnosis of Bordetella pertussis infection. BMC Infect Dis. 2006;6:62. , , , .
- Evaluation of three real‐time PCR assays for detection of Mycoplasma pneumoniae in an outbreak investigation. J Clin Microbiol. 2008;46(9):3116–3118. , , , , .
- Normal ranges of heart rate and respiratory rate in children from birth to 18 years of age: a systematic review of observational studies. Lancet. 2011;377(9770):1011–1018. , , , et al.
- Development of heart and respiratory rate percentile curves for hospitalized children. Pediatrics. 2013;131(4):e1150–e1157. , , , , , .
- Effect of oxygen supplementation on length of stay for infants hospitalized with acute viral bronchiolitis. Pediatrics. 2008;121(3):470–475. , .
- Longitudinal assessment of hemoglobin oxygen saturation in healthy infants during the first 6 months of age. Collaborative Home Infant Monitoring Evaluation (CHIME) Study Group. J Pediatr. 1999;135(5):580–586. , , , et al.
- Prospective multicenter study of bronchiolitis: predicting safe discharges from the emergency department. Pediatrics. 2008;121(4):680–688. , , , et al.
- Delays in discharge in a tertiary care pediatric hospital. J Hosp Med. 2009;4(8):481–485. , , , et al.
- Using quality improvement to optimise paediatric discharge efficiency. BMJ Qual Saf. 2014;23(5):428–436. , , , et al.
- Bronchiolitis in infants and children: treatment; outcome; and prevention. In: Torchia M, ed. UpToDate. Alphen aan den Rijn, the Netherlands; Wolters Kluwer Health; 2013. , .
- Duration of illness in infants with bronchiolitis evaluated in the emergency department. Pediatrics. 2010;126(2):285–290. , .
- Duration of illness in ambulatory children diagnosed with bronchiolitis. Arch Pediatr Adolesc Med. 2000;154(10):997–1000. , , .
- A clinical pathway for bronchiolitis is effective in reducing readmission rates. J Pediatr. 2005;147(5):622–626. , , , et al.
- Measuring hospital quality using pediatric readmission and revisit rates. Pediatrics. 2013;132(3):429–436. , , , et al.
- Pediatric readmission prevalence and variability across hospitals. JAMA. 2013;309(4):372–380. , , , et al.
- Preventability of early readmissions at a children's hospital. Pediatrics. 2013;131(1):e171–e181. , , , , , .
- Hospital readmission: quality indicator or statistical inevitability? Pediatrics. 2013;132(3):569–570. , .
- Children's hospitals with shorter lengths of stay do not have higher readmission rates. J Pediatr. 2013;163(4):1034–1038.e1. , , , et al.
- Trends in bronchiolitis hospitalizations in the United States, 2000–2009. Pediatrics. 2013;132(1):28–36. , , , , .
- Preventable adverse events in infants hospitalized with bronchiolitis. Pediatrics. 2005;116(3):603–608. , , , .
- Infectious disease hospitalizations among infants in the United States. Pediatrics. 2008;121(2):244–252. , , , , .
- “A hospital is no place to be sick” Samuel Goldwyn (1882–1974). Arch Dis Child. 2009;94(8):565–566. .
- Variation in inpatient diagnostic testing and management of bronchiolitis. Pediatrics. 2005;115(4):878–884. , , , , ,
- International variation in the management of infants hospitalized with respiratory syncytial virus. International RSV Study Group. Eur J Pediatr. 1998;157(3):215–220. , , ,
- Population variation in admission rates and duration of inpatient stay for bronchiolitis in England. Arch Dis Child. 2013;98(1):57–59. , , , , .
- Impact of pulse oximetry and oxygen therapy on length of stay in bronchiolitis hospitalizations. Arch Pediatr Adolesc Med. 2004;158(6):527–530. , , , .
- Pulse oximetry in pediatric practice. Pediatrics. 2011;128(4):740–752. , , .
- Scottish Intercollegiate Guidelines Network. Bronchiolitis in children (SIGN 91). In: NHS Quality Improvement Scotland. Edinburgh, Scotland: Scottish Intercollegiate Guidelines Network; 2006.
- Diagnosis and management of bronchiolitis. Pediatrics. 2006;118(4):1774–1793. , , , et al.
- Prospective multicenter study of children with bronchiolitis requiring mechanical ventilation. Pediatrics. 2012;130(3):e492–e500. , , , et al.
- Prospective multicenter study of viral etiology and hospital length of stay in children with severe bronchiolitis. Arch Pediatr Adolesc Med. 2012;166(8):700–706. , , , et al.
- Apnea in children hospitalized with bronchiolitis. Pediatrics. 2013;132(5):e1194–e1201. , , , et al.
- Evaluation of the cardiovascular system: history and physical evaluation. In: Kliegman RM, Stanton BF, St. Geme JW III, Schor NF, Behrman RF, eds. Nelson Textbook of Pediatrics. Philadelphia, PA: Elsevier Saunders; 2011:1529–1536. .
- Apnea in children hospitalized with bronchiolitis. Pediatrics. 2013;132(5):e1194–e1201. , , , et al.
- Respiratory viral infections in patients with chronic, obstructive pulmonary disease. J Infect. 2005;50(4):322–330. , , , et al.
- Evaluation of real‐time PCR for diagnosis of Bordetella pertussis infection. BMC Infect Dis. 2006;6:62. , , , .
- Evaluation of three real‐time PCR assays for detection of Mycoplasma pneumoniae in an outbreak investigation. J Clin Microbiol. 2008;46(9):3116–3118. , , , , .
- Normal ranges of heart rate and respiratory rate in children from birth to 18 years of age: a systematic review of observational studies. Lancet. 2011;377(9770):1011–1018. , , , et al.
- Development of heart and respiratory rate percentile curves for hospitalized children. Pediatrics. 2013;131(4):e1150–e1157. , , , , , .
- Effect of oxygen supplementation on length of stay for infants hospitalized with acute viral bronchiolitis. Pediatrics. 2008;121(3):470–475. , .
- Longitudinal assessment of hemoglobin oxygen saturation in healthy infants during the first 6 months of age. Collaborative Home Infant Monitoring Evaluation (CHIME) Study Group. J Pediatr. 1999;135(5):580–586. , , , et al.
- Prospective multicenter study of bronchiolitis: predicting safe discharges from the emergency department. Pediatrics. 2008;121(4):680–688. , , , et al.
- Delays in discharge in a tertiary care pediatric hospital. J Hosp Med. 2009;4(8):481–485. , , , et al.
- Using quality improvement to optimise paediatric discharge efficiency. BMJ Qual Saf. 2014;23(5):428–436. , , , et al.
- Bronchiolitis in infants and children: treatment; outcome; and prevention. In: Torchia M, ed. UpToDate. Alphen aan den Rijn, the Netherlands; Wolters Kluwer Health; 2013. , .
- Duration of illness in infants with bronchiolitis evaluated in the emergency department. Pediatrics. 2010;126(2):285–290. , .
- Duration of illness in ambulatory children diagnosed with bronchiolitis. Arch Pediatr Adolesc Med. 2000;154(10):997–1000. , , .
- A clinical pathway for bronchiolitis is effective in reducing readmission rates. J Pediatr. 2005;147(5):622–626. , , , et al.
- Measuring hospital quality using pediatric readmission and revisit rates. Pediatrics. 2013;132(3):429–436. , , , et al.
- Pediatric readmission prevalence and variability across hospitals. JAMA. 2013;309(4):372–380. , , , et al.
- Preventability of early readmissions at a children's hospital. Pediatrics. 2013;131(1):e171–e181. , , , , , .
- Hospital readmission: quality indicator or statistical inevitability? Pediatrics. 2013;132(3):569–570. , .
- Children's hospitals with shorter lengths of stay do not have higher readmission rates. J Pediatr. 2013;163(4):1034–1038.e1. , , , et al.
- Trends in bronchiolitis hospitalizations in the United States, 2000–2009. Pediatrics. 2013;132(1):28–36. , , , , .
- Preventable adverse events in infants hospitalized with bronchiolitis. Pediatrics. 2005;116(3):603–608. , , , .
© 2015 Society of Hospital Medicine
IV Antibiotic Duration in Children
Rationally defining the appropriate duration of intravenous (IV) antibiotics for children with bacterial infections is challenging. For example, how long should a 2‐week‐old infant with a urinary tract infection (UTI) caused by Escherichia coli (E coli) be treated intravenously if the infant has responded to treatment and is back to baseline within 1 to 2 days? What if the blood culture was also positive for E coli? What are the risks and benefits of continuing IV antibiotics?
Such questions are common for pediatric hospitalists. Bacterial infections remain a relatively frequent cause of pediatric hospitalization, especially in neonates where 5 of the top 10 causes of hospitalizations are related to bacterial infections.[1] For some conditions, children remain hospitalized after clinical improvement simply for ongoing provision of IV antibiotics. Alternatively, some children are discharged home with a peripherally inserted central catheter (PICC) to complete an IV course.
The decision regarding the duration of IV antibiotics varies according to the condition for which the antibiotic is prescribed and often by practitioner or hospital. Many recommendations are numerically based (eg, 10 days for group B Streptococcus [GBS] bacteremia, 21 days for E coli meningitis), without taking into account patient‐level factors such as initial severity or response to therapy. These concrete recommendations may in fact be preferred by some practitioners, as suggested by a former chairman of the Committee on Infectious Disease for the American Academy of Pediatrics (AAP): The Red Book is designed for people who make decisions. It cannot waffle on an issue. It has to make a positive recommendation even if the data are incomplete.[2] A potential downside of this mentality, however, is that some practitioners may then feel obligated to follow these recommendations despite the lack of supportive evidence.
EXTENDING IV ANTIBIOTICS BEYOND CLINICAL RECOVERY
What is the rationale for continuing IV antibiotics in infants whose symptoms have completely resolved? Several factors likely drive these decisions: prevention of recurrences, concerns about bioavailability of enteral antibiotics and patient compliance, adherence to expert recommendations/guidelines, and perhaps a general sense that more is betterthat serious infections and/or their sequelae require more aggressive treatments.
Recurrence of a potentially life‐threatening infection is an understandable concern. Even when symptoms have resolved and there is documented clearance of the infection, such clearance does not necessarily signify that the body has rid itself of the pathogen completely. Some infections are deep seated and may warrant continuing treatment despite apparent recovery. To some, the risks of prolonging IV therapy may seem inconsequential when juxtaposed to a potentially devastating recurrence. However, in many conditions, recurrences may be related to host issues or ongoing exposures rather than inadequate treatment of the original infection. Recurrent UTIs, for example, are more likely in infants with urologic abnormalities,[3] and recurrent GBS bacteremia has been associated with GBS colonization of maternal breast milk and/or maternal mastitis.[4, 5, 6, 7] Although it is tempting to extend IV courses to prevent recurrences, it is not clear that the benefits of such an approach outweigh the risks.
Concerns over enteral absorption and bioavailability are also understandable, especially in young infants. The superior efficacy of IV over oral antibiotics in general is well accepted for many pediatric conditions, and in some cases (eg, septic shock) it would be unethical to perform a head‐to‐head trial. However, the lack of any published trials (to our knowledge) in pediatrics confirming the superiority of IV antibiotics suggests that oral antibiotic absorption is sufficient for many infections. Even in neonates, several studies have demonstrated that therapeutic serum levels are easily reached with oral dosing of amoxicillin in term and preterm neonates.[8, 9]
For the remainder of this review, the published recommendations and available evidence behind the duration of IV therapy are summarized for 4 bacterial infections in children in which IV antibiotic therapy often continues after clinical recovery: meningitis, bacteremia, UTI, and acute osteomyelitis. We conclude by proposing additional considerations for IV antibiotic durations, especially in situations where guidelines and/or evidence are either nonexistent, dated, conflicting, or contrary to evidence from published studies.
BACTERIAL MENINGITIS
The Infectious Disease Society of America and the British National Institute for Clinical Evidence have both published guidelines with pediatric recommendations for duration of therapy in bacterial meningitis,[10, 11] though the recommendations differ somewhat for 3 of the 4 most common pathogens, and are not always concordant with evidence from randomized controlled trials (Table 1).[12, 13, 14]
Pathogen | IDSA | NICE | Minimum Range Achieving Equivalent Outcomes in Recent Randomized Trials |
---|---|---|---|
| |||
Group B Streptococcus | 1421 days | 14 days | None available |
Neisseria meningititis | 7 days | 7 days | 15 days[12, 13, 14] |
Haemophilus influenzae type b | 7 days | 10 days | 45 days[12, 13] |
Streptococcus pneumoniae | 1014 days | 14 days | 45 days[12, 13] |
A recent meta‐analysis on duration of therapy in meningitis included 5 open‐label trials of ceftriaxone for bacterial meningitis in children.[12] These trials included the 3 most common pathogens and were categorized as short‐course (47 days, n=196 patients) and long‐course (714 days, n=187 patients) therapy. There was no significant difference in clinical success or long‐term neurological complications between groups. Subsequently, a multicountry trial enrolled over 1000 children 2 months to 12 years of age with meningitis caused by Haemophilus influenzae type b, Streptococcus pneumonia, or Neisseria meningititis who were stable after 5 days of IV ceftriaxone therapy and randomized them to receive placebo or an additional 5 days of ceftriaxone.[13] Patients with persistence of seizures, bacteremia, abscess or distant infections, or who were judged to be deteriorating or still severely ill at the 5‐day point were excluded (4.7% of the children who were recruited on day 0). There were no significant differences in bacteriologic failures, clinical failures, or clinical sequelae in survivors. The authors concluded that ceftriaxone can be discontinued in children with bacterial meningitis who are clinically stable after 5 days of IV therapy. Further trials in developed countries are needed.
BACTEREMIA
Because of routine vaccination against H influenzae type b and S pneumoniae, bacteremia beyond the first few months of life in otherwise healthy children is now rare.[15] Even in infants too young to benefit directly from vaccination, the epidemiology of bacteremia has changed considerably over the last few decades, with E coli and GBS constituting the majority (65%77%) of cases.[16, 17] We will limit this review on bacteremia to these 2 organisms in young infants.
Most cases of E coli bacteremia are associated with UTI (91%98%),[16, 17] and most bacteremic UTIs (88%92%) are caused by E coli.[18, 19, 20, 21] There are no official recommendations for the duration of treatment of bacteremic UTI, and only a limited amount of evidence can be gleaned from existing studies. In a trial of oral cefixime for infants aged 1 to 24 months with UTI, all 13 infants with bacteremia fared well whether they received oral cefixime only or IV cefotaxime for 3 days followed by oral cefixime.[18] In a study on length of IV antibiotic therapy in over 12,000 infants <6 months old with UTI, the presence of bacteremia predicted longer IV treatment length (bacteremia was present in 0.5% of the short IV group vs 0.8% of the long IV group, P=0.02) but did not predict treatment failure, defined as readmission within 30 days.[3] In a multicenter investigation of 229 infants <3 months old with bacteremic UTI, the duration of parenteral antibiotics was extremely variable (range, 117 days) and was not associated with treatment failure, defined as recurrent UTI caused by the same organism within 30 days (mean duration 7.8 days in the treatment‐failure group vs 7.7 days in the no‐failure group, P=0.99).[21] In summary, there is no evidence to support a prolonged course (ie, >35 days) of IV antibiotics for bacteremic UTI.
For bacteremia caused by GBS, although the Red Book Committee on Infectious Disease recommends 10 days of IV antibiotics,[22] to our knowledge there are no experimental or observational investigations to support this recommendation. Although available studies suggest that IV courses of at least 10 days are generally provided,[7, 23] no studies have compared outcomes of infants treated with short versus long courses. However, in a study that included 29 full‐term neonates with GBS bacteremia, all 29 had responded initially to 48 hours of intravenous antibiotics (defined as being asymptomatic and fed enterally), and were then treated successfully with high‐dose oral amoxicillin for the remainder of the course, with no recurrences.[8] Although recurrences are estimated to occur in 0.5% to 3% of babies treated for GBS infections, many recurrences are associated with exposure factors such as GBS colonization of the breast milk.[4, 5, 6, 7] In summary, although 10 or more days of IV antibiotic therapy remains a common published recommendation, there is no supportive evidence. More research is needed to assess whether shorter IV courses are safe.
UTI
Most UTIs can be treated with oral antibiotics.[24] In its practice parameter on febrile UTIs in infants 2 months to 2 years of age, the AAP recommends oral antibiotics for well‐appearing children.[25] This recommendation is supported by a recent Cochrane review on the topic,[26] and at least 3 additional trials that have demonstrated that long IV courses do not yield better outcomes than shorter IV courses or oral only courses.[27, 28, 29]
However, all of these trials exclude infants <1 month old, and there are no published recommendations for the <2‐month‐old age group. The study by Brady et al. on >12,000 infants <6 months old with UTI demonstrated no significant differences in UTI readmission rates between infants who were given 4 days of IV antibiotics versus those who were given <4 days.[3] There were 3,383 infants <30 days old in this study, and about one‐third of these babies received a short IV course. Failure rates were nearly identical in each group (2.3% in short course vs 2.4% in long course) even after risk adjustment (personal communication with Patrick Brady, MD, on February 7, 2014). Magin et al. describe 172 neonates (median age 19 days) with UTI who were treated intravenously for a median duration of 4 days (interquartile range, 36 days) and did not experience treatment failures or relapses.[30]
In summary, most cases of UTI can be managed with oral antibiotics. Uncertainty remains over the optimal approach for infants <1 to 2 months old, an age range not considered in current published guidelines. Current evidence suggests that IV treatment for 3 to 4 days followed by oral therapy may be sufficient treatment in this age group.
ACUTE OSTEOMYELITIS
Given the excellent blood supply to rapidly growing tissues in children, shorter durations of IV therapy have been studied with increasing frequency. A 2002 systematic review included 12 prospective cohort studies with at least 6 months of follow‐up.[31] Studies were stratified into 7 days or >7 days IV therapy, and there were no differences in cure rates. Subsequently, a large Finnish trial reported on 131 children who received an initial short IV course (24 days) followed by 20 versus 30 total days of therapy with very low treatment failure rates.[32]
The largest study from the United States to date analyzed nearly 2000 cases of osteomyelitis from 29 hospitals.[33] This study defined a prolonged IV course by placement of a central venous catheter. The rates of prolonged IV therapy varied significantly across hospitals, ranging from 10% to 95% of patients, without detectable differences in outcomes. Furthermore, the readmission rate for catheter related complications (3%) was nearly as high as the overall treatment failure rate (4%5%). Recently, Arnold et al. reported 8 years' experience with a management algorithm to guide the transition to oral antibiotics in pediatric osteoarticular infections in a patient specific manner.[34] This study included 194 patients (154 uncomplicated and 40 complicated cases), all with culture‐proven disease. Transition to oral antibiotics occurred based on resolution of fever and pain, improved function of the affected region, and a C‐reactive protein level of <3 mg/dL, and occurred at an average of 10 days into the treatment course. These authors also provided extensive information about complications to demonstrate that the proposed strategy can be used with a wide range of patients and pathogens. There was a single microbiologic treatment failure after oral step‐down therapy in a complicated osteoarticular infection, with a retained bony fragment. This study represents a successful example of a patient‐centered approach to IV antibiotic duration.
A PATIENT‐CENTERED APPROACH
Returning to the example above of the 2‐week‐old with UTI (with or without bacteremia), there are no published guidelines and only limited available evidence to help guide the duration of IV antibiotics in this case. When standards of care (eg, from published guidelines, review articles, textbooks, or local expert guidance) are nonexistent, conflicting, dated, or contrary to existing evidence, patient‐level factors can be incorporated into the decision‐making process (Table 2). In these cases, tailoring the IV antibiotic course to the individual's response (referred to in 1 review as the ultimate bioassay of the therapy[2]), while also weighing risks and benefits of ongoing therapy, is a logical approach.
Consideration | Description |
---|---|
| |
Severity of initial infection | If concern of recurrence is the justification for a longer IV course, then a more prolonged course might be considered for a more severe initial presentation (eg, septic shock, multisystem organ failure, intensive care unit admission). |
Response to therapy | Continued IV antibiotics might be warranted in patients who are still symptomatic (eg, fever, vomiting). Inflammatory markers have been used to guide therapy in osteomyelitis.[34] |
Patient compliance | If a child does not tolerate oral antibiotics or there are concerns about family adherence, a longer IV course may be considered. |
Family preferences | Shared decision making can be employed, especially when there is no clear evidence supporting a specific duration. |
Assessment of harms of ongoing hospitalization and/or prolonged IV therapy | See Table 3 |
SEVERITY OF INITIAL INFECTION AND RESPONSE TO THERAPY
The severity of the initial infection, whether in terms of presentation or clinical recovery, can factor into the duration of therapy. Provision of a longer IV course to prevent (albeit theoretically) a recurrence makes more logical sense in an infant with GBS bacteremia who was ill enough to warrant intensive care unit admission than in an infant whose only symptom was a fever. Similarly, most practitioners would be reluctant to stop IV antibiotics and discharge a patient with a bacterial infection who is persistently febrile or vomiting. Although the use of inflammatory markers and other clinical symptoms to guide therapy has been limited to osteomyelitis, this approach might be useful and should be studied in other conditions.
SHARED DECISION MAKING
Shared decision making can also be employed. Parents of sick, hospitalized children generally prefer to be involved in the decision‐making process.[35] For a parent who has concerns about their child's well‐being in the hospital, or has multiple other children at home, competing career obligations, and/or limited family support, the burden of ongoing hospitalization can be significant, and should be factored into decision making. Involving parents in medical decisions may lead to a reduction in utilization for some conditions.[36]
ASSESSMENT OF RISKS/COSTS
The risks and costs of pediatric hospitalization and prolonged IV antibiotics are well described in the literature and are summarized in Table 3. Although the benefits of prolonging IV antibiotics in a child who has recovered from an acute bacterial infection are largely theoretical, many of the risks are concrete and quantifiable. For example, a young infant being treated for a bacteremic UTI may run out of potential IV sites and need a PICC line to continue IV therapy, which according to a recent review of 2574 PICC lines has a 21% complication rate. This rate is even higher in children for whom the PICC line indication was provision of antibiotics (27%) and for infants <1 year of age (44%).[37] Moreover, this procedure often requires sedation or anesthesia for placement, which has both known and unknown risks, including concerns about subsequent adverse effects on development in young children.[38] Nosocomial exposure to seasonal viruses poses an additional risk to hospitalized children.[39]
Harm of Intravenous Antibiotic Therapy | Description or Example |
---|---|
| |
Complications from peripheral IV catheter | Leading source of pain and distress for hospitalized children.[44] |
Serious complications can occur following IV infiltrates.[45] | |
Complications from PICC line | Approximately 20% overall complication rate (44% in infants <1 year old).[37] |
Complications led to rehospitalization of 3% of children being treated with prolonged antibiotics for osteomyelitis.[33] | |
When thrombosis occurs (up to 9% risk in neonates[46]), 3 months of anticoagulation is recommended.[47] | |
Complications may arise from sedation/anesthesia necessary to place catheter. Anesthesia has been associated with adverse behavioral or developmental outcomes in children <4 years of age.[38] | |
Risk of nosocomial infection while hospitalized | An estimated 6% of hospital RSV infections are nosocomial, which are associated with a more prolonged LOS than hospitalizations for community‐acquired RSV.[39] |
Medication error | In 1 investigation, serious medication errors occurred in 22 per 1,000 patient‐days in a large children's hospital.[48] |
Emotional and financial burdens | Hospitalization can pose a significant strain on the child, parents, and siblings. |
Financial costs to healthcare system | In 2003, infection‐related hospitalizations in infants had an average cost of $4,000 (average LOS 3.5 days).[1] |
Harms associated with prolonged courses of antibiotics in general (IV or PO) | Antibiotic resistance, diarrhea (including Clostridium difficile), allergic reactions, increased costs.[49] |
These additional considerations for the duration of IV antibiotics are not evidence based and should not be used to justify an IV duration that differs dramatically from an accepted standard of care. These are merely considerations that incorporate clinical judgment and a comprehensive analysis of risks and benefits in situations where the available evidence is suboptimal. This approach can be adopted both as a framework for future research and directly in clinical practice.
CONCLUSION
In an era of increasing focus on overtreatment/waste,[40] patient safety,[41] and patient‐centered care,[42] the duration of IV antibiotics for common bacterial infections is a prime target for improving pediatric healthcare value. As emphasized by Michael Porter recently in The New England Journal of Medicine, value should always be defined around the customer.[43] A high‐value approach to IV antibiotic duration incorporates a rigorous assessment of risks and benefits that focuses on best evidence and patient‐level factors.
In discussing published guidelines in a review on bacterial meningitis therapy, Michael Radetsky noted that [R]ecommended criteria, even if provisional, may inadvertently become invested with an independent power to force submission and prohibit deviation. The danger is that sensitivity to individual responsiveness and variability will be lost.[2] Guidelines are useful tools in pediatrics and should continue to be used to direct IV antibiotic durations for bacterial infections in children. However, the emphasis on fixed durations of IV antibiotics might not always serve the best interest of the patient. When guidelines are lacking or contradictory, patient factors should also be considered.
Acknowledgements
The authors thank Ellen R. Wald, MD, and Kenneth B. Roberts, MD, for their thoughtful and valuable additions to this review.
Disclosure: Nothing to report.
- Infectious disease hospitalizations among infants in the United States. Pediatrics. 2008;121(2):244–252. , , , , .
- Duration of treatment in bacterial meningitis: a historical inquiry. Pediatr Infect Dis J. 1990;9(1):2–9. .
- Length of intravenous antibiotic therapy and treatment failure in infants with urinary tract infections. Pediatrics. 2010;126(2):196–203. , , .
- Group B streptococci in milk and late neonatal infections: an analysis of cases in the literature. Arch Dis Child Fetal Neonatal Ed. 2014;99(1):F41–F47. , , , et al.
- Recurrent late‐onset group B Streptococcus sepsis in a preterm infant acquired by expressed breastmilk transmission: a case report. Breastfeed Med. 2013;8(1):134–136. , , .
- Late‐onset and recurrent neonatal Group B streptococcal disease associated with breast‐milk transmission. Pediatr Dev Pathol. 2003;6(3):251–256. , , , , , .
- A 5‐year review of recurrent group B streptococcal disease: lessons from twin infants. Clin Infect Dis. 2000;30(2):282–287. , , , , .
- Therapeutic amoxicillin levels achieved with oral administration in term neonates. Eur J Clin Pharmacol. 2007;63(7):657–662. , , , et al.
- Population pharmacokinetics and dosing of amoxicillin in (pre)term neonates. Ther Drug Monit. 2006;28(2):226–231. , , , , , .
- Practice guidelines for the management of bacterial meningitis. Clin Infect Dis. 2004;39(9):1267–1284. , , , et al.
- Management of bacterial meningitis and meningococcal septicaemia in children and young people: summary of NICE guidance. BMJ. 2010;340:c3209. , , , , , .
- Short versus long duration of antibiotic therapy for bacterial meningitis: a meta‐analysis of randomised controlled trials in children. Arch Dis Child. 2009;94(8):607–614. , , , , .
- 5 versus 10 days of treatment with ceftriaxone for bacterial meningitis in children: a double‐blind randomised equivalence study. Lancet. 2011;377(9780):1837–1845. , , , et al.
- Ceftriaxone as effective as long‐acting chloramphenicol in short‐course treatment of meningococcal meningitis during epidemics: a randomised non‐inferiority study. Lancet. 2005;366(9482):308–313. , , , et al.
- Changing epidemiology of outpatient bacteremia in 3‐ to 36‐month‐old children after the introduction of the heptavalent‐conjugated pneumococcal vaccine. Pediatr Infect Dis J. 2006;25(4):293–300. , , , et al.
- Epidemiology of bacteremia in febrile infants in the United States. Pediatrics. 2013;132(6):990–996. , , , et al.
- Changing epidemiology of bacteremia in infants aged 1 week to 3 months. Pediatrics. 2012;129(3):e590–e596. , , .
- Oral versus initial intravenous therapy for urinary tract infections in young febrile children. Pediatrics. 1999;104(1 pt 1):79–86. , , , et al.
- Bacteremic urinary tract infection in children. Pediatr Infect Dis J. 2000;19(7):630–634. , , , , .
- Urine testing and urinary tract infections in febrile infants seen in office settings: the Pediatric Research in Office Settings' Febrile Infant Study. Arch Pediatr Adolesc Med. 2002;156(1):44–54. , , , , , .
- 2014; Vancouver BC, Canada. , , , , , . Management of bacteremic urinary tract infections in infants less than 3 months of age. Abstract presented at: Pediatric Academic Societies Annual Meeting; May 5,
- Red Book: 2009 Report of the Committee on Infectious Diseases. 28th ed. Elk Grove Village, IL: American Academy of Pediatrics; 2012. , , , .
- Recurrent group B streptococcal bacteremia. Clin Pediatr (Phila). 2012;51(9):884–887. , .
- Antibiotics for treating lower urinary tract infection in children. Cochrane Database Syst Rev. 2012;8:CD006857. , , , .
- Urinary tract infection: clinical practice guideline for the diagnosis and management of the initial UTI in febrile infants and children 2 to 24 months. Pediatrics. 2011;128(3):595–610. .
- Antibiotics for acute pyelonephritis in children. Cochrane Database Syst Rev. 2007(4):CD003772. , , .
- Randomized trial of oral versus sequential IV/oral antibiotic for acute pyelonephritis in children. Pediatrics. 2012;129(2):e269–e275. , , , et al.
- Prospective, randomized trial comparing short and long intravenous antibiotic treatment of acute pyelonephritis in children: dimercaptosuccinic acid scintigraphic evaluation at 9 months. Pediatrics. 2008;121(3):e553–e560. , , , et al.
- Randomised trial of oral versus sequential intravenous/oral cephalosporins in children with pyelonephritis. Eur J Pediatr. 2008;167(9):1037–1047. , , , et al.
- Efficacy of short‐term intravenous antibiotic in neonates with urinary tract infection. Pediatr Emerg Care. 2007;23(2):83–86. , , , , .
- Shorter courses of parenteral antibiotic therapy do not appear to influence response rates for children with acute hematogenous osteomyelitis: a systematic review. BMC Infect Dis. 2002;2:16. , , , , , .
- Short‐ versus long‐term antimicrobial treatment for acute hematogenous osteomyelitis of childhood: prospective, randomized trial on 131 culture‐positive cases. Pediatr Infect Dis J. 2010;29(12):1123–1128. , , , .
- Prolonged intravenous therapy versus early transition to oral antimicrobial therapy for acute osteomyelitis in children. Pediatrics. 2009;123(2):636–642. , , , , , .
- Acute bacterial osteoarticular infections: eight‐year analysis of C‐reactive protein for oral step‐down therapy. Pediatrics. 2012;130(4):e821–e828. , , , et al.
- Parental decision‐making preferences in the pediatric intensive care unit. Crit Care Med. 2012;40(10):2876–2882. , , , , , .
- An assessment of the shared‐decision model in parents of children with acute otitis media. Pediatrics. 2005;116(6):1267–1275. , , , , .
- Risk factors for peripherally inserted central venous catheter complications in children. JAMA Pediatr. 2013;167(5):429–435. , , , , .
- Current clinical evidence on the effect of general anesthesia on neurodevelopment in children: an updated systematic review with meta‐regression. PLoS One. 2014;9(1):e85760. , , .
- Nosocomial respiratory syncytial virus infection in Canadian pediatric hospitals: a Pediatric Investigators Collaborative Network on Infections in Canada Study. Pediatrics. 1997;100(6):943–946. , , , et al.
- Eliminating waste in US health care. JAMA. 2012;307(14):1513–1516. , .
- Safely doing less: a missing component of the patient safety dialogue. Pediatrics. 2011;128(6):e1596–e1597. , , .
- Committee On Hospital Care and Institute For Patient‐ and Family‐Centered Care. Patient‐ and family‐centered care and the pediatrician's role. Pediatrics. 2012;129(2):394–404.
- What is value in health care? N Engl J Med. 2010;363(26):2477–2481. .
- Prevalence and source of pain in pediatric inpatients. Pain. 1996;68(1):25–31. , , , , .
- Acute compartment syndrome of the upper extremity in children: diagnosis, management, and outcomes. J Child Orthop. 2013;7(3):225–233. , , , .
- Neonatal central venous catheter thrombosis: diagnosis, management and outcome. Blood Coagul Fibrinolysis. 2014;25(2):97–106. , , , , .
- Antithrombotic therapy in neonates and children: antithrombotic therapy and prevention of thrombosis, 9th ed: American C ollege of Chest Physicians Evidence‐Based Clinical Practice Guidelines. Chest. 2012;141(2 suppl):e737S–801S. , , , et al.
- Effect of computer order entry on prevention of serious medication errors in hospitalized children. Pediatrics. 2008;121(3):e421–e427. , , , et al.
- Duration and cessation of antimicrobial treatment. J Hosp Med. 2012;7(suppl 1):S22–S33.
Rationally defining the appropriate duration of intravenous (IV) antibiotics for children with bacterial infections is challenging. For example, how long should a 2‐week‐old infant with a urinary tract infection (UTI) caused by Escherichia coli (E coli) be treated intravenously if the infant has responded to treatment and is back to baseline within 1 to 2 days? What if the blood culture was also positive for E coli? What are the risks and benefits of continuing IV antibiotics?
Such questions are common for pediatric hospitalists. Bacterial infections remain a relatively frequent cause of pediatric hospitalization, especially in neonates where 5 of the top 10 causes of hospitalizations are related to bacterial infections.[1] For some conditions, children remain hospitalized after clinical improvement simply for ongoing provision of IV antibiotics. Alternatively, some children are discharged home with a peripherally inserted central catheter (PICC) to complete an IV course.
The decision regarding the duration of IV antibiotics varies according to the condition for which the antibiotic is prescribed and often by practitioner or hospital. Many recommendations are numerically based (eg, 10 days for group B Streptococcus [GBS] bacteremia, 21 days for E coli meningitis), without taking into account patient‐level factors such as initial severity or response to therapy. These concrete recommendations may in fact be preferred by some practitioners, as suggested by a former chairman of the Committee on Infectious Disease for the American Academy of Pediatrics (AAP): The Red Book is designed for people who make decisions. It cannot waffle on an issue. It has to make a positive recommendation even if the data are incomplete.[2] A potential downside of this mentality, however, is that some practitioners may then feel obligated to follow these recommendations despite the lack of supportive evidence.
EXTENDING IV ANTIBIOTICS BEYOND CLINICAL RECOVERY
What is the rationale for continuing IV antibiotics in infants whose symptoms have completely resolved? Several factors likely drive these decisions: prevention of recurrences, concerns about bioavailability of enteral antibiotics and patient compliance, adherence to expert recommendations/guidelines, and perhaps a general sense that more is betterthat serious infections and/or their sequelae require more aggressive treatments.
Recurrence of a potentially life‐threatening infection is an understandable concern. Even when symptoms have resolved and there is documented clearance of the infection, such clearance does not necessarily signify that the body has rid itself of the pathogen completely. Some infections are deep seated and may warrant continuing treatment despite apparent recovery. To some, the risks of prolonging IV therapy may seem inconsequential when juxtaposed to a potentially devastating recurrence. However, in many conditions, recurrences may be related to host issues or ongoing exposures rather than inadequate treatment of the original infection. Recurrent UTIs, for example, are more likely in infants with urologic abnormalities,[3] and recurrent GBS bacteremia has been associated with GBS colonization of maternal breast milk and/or maternal mastitis.[4, 5, 6, 7] Although it is tempting to extend IV courses to prevent recurrences, it is not clear that the benefits of such an approach outweigh the risks.
Concerns over enteral absorption and bioavailability are also understandable, especially in young infants. The superior efficacy of IV over oral antibiotics in general is well accepted for many pediatric conditions, and in some cases (eg, septic shock) it would be unethical to perform a head‐to‐head trial. However, the lack of any published trials (to our knowledge) in pediatrics confirming the superiority of IV antibiotics suggests that oral antibiotic absorption is sufficient for many infections. Even in neonates, several studies have demonstrated that therapeutic serum levels are easily reached with oral dosing of amoxicillin in term and preterm neonates.[8, 9]
For the remainder of this review, the published recommendations and available evidence behind the duration of IV therapy are summarized for 4 bacterial infections in children in which IV antibiotic therapy often continues after clinical recovery: meningitis, bacteremia, UTI, and acute osteomyelitis. We conclude by proposing additional considerations for IV antibiotic durations, especially in situations where guidelines and/or evidence are either nonexistent, dated, conflicting, or contrary to evidence from published studies.
BACTERIAL MENINGITIS
The Infectious Disease Society of America and the British National Institute for Clinical Evidence have both published guidelines with pediatric recommendations for duration of therapy in bacterial meningitis,[10, 11] though the recommendations differ somewhat for 3 of the 4 most common pathogens, and are not always concordant with evidence from randomized controlled trials (Table 1).[12, 13, 14]
Pathogen | IDSA | NICE | Minimum Range Achieving Equivalent Outcomes in Recent Randomized Trials |
---|---|---|---|
| |||
Group B Streptococcus | 1421 days | 14 days | None available |
Neisseria meningititis | 7 days | 7 days | 15 days[12, 13, 14] |
Haemophilus influenzae type b | 7 days | 10 days | 45 days[12, 13] |
Streptococcus pneumoniae | 1014 days | 14 days | 45 days[12, 13] |
A recent meta‐analysis on duration of therapy in meningitis included 5 open‐label trials of ceftriaxone for bacterial meningitis in children.[12] These trials included the 3 most common pathogens and were categorized as short‐course (47 days, n=196 patients) and long‐course (714 days, n=187 patients) therapy. There was no significant difference in clinical success or long‐term neurological complications between groups. Subsequently, a multicountry trial enrolled over 1000 children 2 months to 12 years of age with meningitis caused by Haemophilus influenzae type b, Streptococcus pneumonia, or Neisseria meningititis who were stable after 5 days of IV ceftriaxone therapy and randomized them to receive placebo or an additional 5 days of ceftriaxone.[13] Patients with persistence of seizures, bacteremia, abscess or distant infections, or who were judged to be deteriorating or still severely ill at the 5‐day point were excluded (4.7% of the children who were recruited on day 0). There were no significant differences in bacteriologic failures, clinical failures, or clinical sequelae in survivors. The authors concluded that ceftriaxone can be discontinued in children with bacterial meningitis who are clinically stable after 5 days of IV therapy. Further trials in developed countries are needed.
BACTEREMIA
Because of routine vaccination against H influenzae type b and S pneumoniae, bacteremia beyond the first few months of life in otherwise healthy children is now rare.[15] Even in infants too young to benefit directly from vaccination, the epidemiology of bacteremia has changed considerably over the last few decades, with E coli and GBS constituting the majority (65%77%) of cases.[16, 17] We will limit this review on bacteremia to these 2 organisms in young infants.
Most cases of E coli bacteremia are associated with UTI (91%98%),[16, 17] and most bacteremic UTIs (88%92%) are caused by E coli.[18, 19, 20, 21] There are no official recommendations for the duration of treatment of bacteremic UTI, and only a limited amount of evidence can be gleaned from existing studies. In a trial of oral cefixime for infants aged 1 to 24 months with UTI, all 13 infants with bacteremia fared well whether they received oral cefixime only or IV cefotaxime for 3 days followed by oral cefixime.[18] In a study on length of IV antibiotic therapy in over 12,000 infants <6 months old with UTI, the presence of bacteremia predicted longer IV treatment length (bacteremia was present in 0.5% of the short IV group vs 0.8% of the long IV group, P=0.02) but did not predict treatment failure, defined as readmission within 30 days.[3] In a multicenter investigation of 229 infants <3 months old with bacteremic UTI, the duration of parenteral antibiotics was extremely variable (range, 117 days) and was not associated with treatment failure, defined as recurrent UTI caused by the same organism within 30 days (mean duration 7.8 days in the treatment‐failure group vs 7.7 days in the no‐failure group, P=0.99).[21] In summary, there is no evidence to support a prolonged course (ie, >35 days) of IV antibiotics for bacteremic UTI.
For bacteremia caused by GBS, although the Red Book Committee on Infectious Disease recommends 10 days of IV antibiotics,[22] to our knowledge there are no experimental or observational investigations to support this recommendation. Although available studies suggest that IV courses of at least 10 days are generally provided,[7, 23] no studies have compared outcomes of infants treated with short versus long courses. However, in a study that included 29 full‐term neonates with GBS bacteremia, all 29 had responded initially to 48 hours of intravenous antibiotics (defined as being asymptomatic and fed enterally), and were then treated successfully with high‐dose oral amoxicillin for the remainder of the course, with no recurrences.[8] Although recurrences are estimated to occur in 0.5% to 3% of babies treated for GBS infections, many recurrences are associated with exposure factors such as GBS colonization of the breast milk.[4, 5, 6, 7] In summary, although 10 or more days of IV antibiotic therapy remains a common published recommendation, there is no supportive evidence. More research is needed to assess whether shorter IV courses are safe.
UTI
Most UTIs can be treated with oral antibiotics.[24] In its practice parameter on febrile UTIs in infants 2 months to 2 years of age, the AAP recommends oral antibiotics for well‐appearing children.[25] This recommendation is supported by a recent Cochrane review on the topic,[26] and at least 3 additional trials that have demonstrated that long IV courses do not yield better outcomes than shorter IV courses or oral only courses.[27, 28, 29]
However, all of these trials exclude infants <1 month old, and there are no published recommendations for the <2‐month‐old age group. The study by Brady et al. on >12,000 infants <6 months old with UTI demonstrated no significant differences in UTI readmission rates between infants who were given 4 days of IV antibiotics versus those who were given <4 days.[3] There were 3,383 infants <30 days old in this study, and about one‐third of these babies received a short IV course. Failure rates were nearly identical in each group (2.3% in short course vs 2.4% in long course) even after risk adjustment (personal communication with Patrick Brady, MD, on February 7, 2014). Magin et al. describe 172 neonates (median age 19 days) with UTI who were treated intravenously for a median duration of 4 days (interquartile range, 36 days) and did not experience treatment failures or relapses.[30]
In summary, most cases of UTI can be managed with oral antibiotics. Uncertainty remains over the optimal approach for infants <1 to 2 months old, an age range not considered in current published guidelines. Current evidence suggests that IV treatment for 3 to 4 days followed by oral therapy may be sufficient treatment in this age group.
ACUTE OSTEOMYELITIS
Given the excellent blood supply to rapidly growing tissues in children, shorter durations of IV therapy have been studied with increasing frequency. A 2002 systematic review included 12 prospective cohort studies with at least 6 months of follow‐up.[31] Studies were stratified into 7 days or >7 days IV therapy, and there were no differences in cure rates. Subsequently, a large Finnish trial reported on 131 children who received an initial short IV course (24 days) followed by 20 versus 30 total days of therapy with very low treatment failure rates.[32]
The largest study from the United States to date analyzed nearly 2000 cases of osteomyelitis from 29 hospitals.[33] This study defined a prolonged IV course by placement of a central venous catheter. The rates of prolonged IV therapy varied significantly across hospitals, ranging from 10% to 95% of patients, without detectable differences in outcomes. Furthermore, the readmission rate for catheter related complications (3%) was nearly as high as the overall treatment failure rate (4%5%). Recently, Arnold et al. reported 8 years' experience with a management algorithm to guide the transition to oral antibiotics in pediatric osteoarticular infections in a patient specific manner.[34] This study included 194 patients (154 uncomplicated and 40 complicated cases), all with culture‐proven disease. Transition to oral antibiotics occurred based on resolution of fever and pain, improved function of the affected region, and a C‐reactive protein level of <3 mg/dL, and occurred at an average of 10 days into the treatment course. These authors also provided extensive information about complications to demonstrate that the proposed strategy can be used with a wide range of patients and pathogens. There was a single microbiologic treatment failure after oral step‐down therapy in a complicated osteoarticular infection, with a retained bony fragment. This study represents a successful example of a patient‐centered approach to IV antibiotic duration.
A PATIENT‐CENTERED APPROACH
Returning to the example above of the 2‐week‐old with UTI (with or without bacteremia), there are no published guidelines and only limited available evidence to help guide the duration of IV antibiotics in this case. When standards of care (eg, from published guidelines, review articles, textbooks, or local expert guidance) are nonexistent, conflicting, dated, or contrary to existing evidence, patient‐level factors can be incorporated into the decision‐making process (Table 2). In these cases, tailoring the IV antibiotic course to the individual's response (referred to in 1 review as the ultimate bioassay of the therapy[2]), while also weighing risks and benefits of ongoing therapy, is a logical approach.
Consideration | Description |
---|---|
| |
Severity of initial infection | If concern of recurrence is the justification for a longer IV course, then a more prolonged course might be considered for a more severe initial presentation (eg, septic shock, multisystem organ failure, intensive care unit admission). |
Response to therapy | Continued IV antibiotics might be warranted in patients who are still symptomatic (eg, fever, vomiting). Inflammatory markers have been used to guide therapy in osteomyelitis.[34] |
Patient compliance | If a child does not tolerate oral antibiotics or there are concerns about family adherence, a longer IV course may be considered. |
Family preferences | Shared decision making can be employed, especially when there is no clear evidence supporting a specific duration. |
Assessment of harms of ongoing hospitalization and/or prolonged IV therapy | See Table 3 |
SEVERITY OF INITIAL INFECTION AND RESPONSE TO THERAPY
The severity of the initial infection, whether in terms of presentation or clinical recovery, can factor into the duration of therapy. Provision of a longer IV course to prevent (albeit theoretically) a recurrence makes more logical sense in an infant with GBS bacteremia who was ill enough to warrant intensive care unit admission than in an infant whose only symptom was a fever. Similarly, most practitioners would be reluctant to stop IV antibiotics and discharge a patient with a bacterial infection who is persistently febrile or vomiting. Although the use of inflammatory markers and other clinical symptoms to guide therapy has been limited to osteomyelitis, this approach might be useful and should be studied in other conditions.
SHARED DECISION MAKING
Shared decision making can also be employed. Parents of sick, hospitalized children generally prefer to be involved in the decision‐making process.[35] For a parent who has concerns about their child's well‐being in the hospital, or has multiple other children at home, competing career obligations, and/or limited family support, the burden of ongoing hospitalization can be significant, and should be factored into decision making. Involving parents in medical decisions may lead to a reduction in utilization for some conditions.[36]
ASSESSMENT OF RISKS/COSTS
The risks and costs of pediatric hospitalization and prolonged IV antibiotics are well described in the literature and are summarized in Table 3. Although the benefits of prolonging IV antibiotics in a child who has recovered from an acute bacterial infection are largely theoretical, many of the risks are concrete and quantifiable. For example, a young infant being treated for a bacteremic UTI may run out of potential IV sites and need a PICC line to continue IV therapy, which according to a recent review of 2574 PICC lines has a 21% complication rate. This rate is even higher in children for whom the PICC line indication was provision of antibiotics (27%) and for infants <1 year of age (44%).[37] Moreover, this procedure often requires sedation or anesthesia for placement, which has both known and unknown risks, including concerns about subsequent adverse effects on development in young children.[38] Nosocomial exposure to seasonal viruses poses an additional risk to hospitalized children.[39]
Harm of Intravenous Antibiotic Therapy | Description or Example |
---|---|
| |
Complications from peripheral IV catheter | Leading source of pain and distress for hospitalized children.[44] |
Serious complications can occur following IV infiltrates.[45] | |
Complications from PICC line | Approximately 20% overall complication rate (44% in infants <1 year old).[37] |
Complications led to rehospitalization of 3% of children being treated with prolonged antibiotics for osteomyelitis.[33] | |
When thrombosis occurs (up to 9% risk in neonates[46]), 3 months of anticoagulation is recommended.[47] | |
Complications may arise from sedation/anesthesia necessary to place catheter. Anesthesia has been associated with adverse behavioral or developmental outcomes in children <4 years of age.[38] | |
Risk of nosocomial infection while hospitalized | An estimated 6% of hospital RSV infections are nosocomial, which are associated with a more prolonged LOS than hospitalizations for community‐acquired RSV.[39] |
Medication error | In 1 investigation, serious medication errors occurred in 22 per 1,000 patient‐days in a large children's hospital.[48] |
Emotional and financial burdens | Hospitalization can pose a significant strain on the child, parents, and siblings. |
Financial costs to healthcare system | In 2003, infection‐related hospitalizations in infants had an average cost of $4,000 (average LOS 3.5 days).[1] |
Harms associated with prolonged courses of antibiotics in general (IV or PO) | Antibiotic resistance, diarrhea (including Clostridium difficile), allergic reactions, increased costs.[49] |
These additional considerations for the duration of IV antibiotics are not evidence based and should not be used to justify an IV duration that differs dramatically from an accepted standard of care. These are merely considerations that incorporate clinical judgment and a comprehensive analysis of risks and benefits in situations where the available evidence is suboptimal. This approach can be adopted both as a framework for future research and directly in clinical practice.
CONCLUSION
In an era of increasing focus on overtreatment/waste,[40] patient safety,[41] and patient‐centered care,[42] the duration of IV antibiotics for common bacterial infections is a prime target for improving pediatric healthcare value. As emphasized by Michael Porter recently in The New England Journal of Medicine, value should always be defined around the customer.[43] A high‐value approach to IV antibiotic duration incorporates a rigorous assessment of risks and benefits that focuses on best evidence and patient‐level factors.
In discussing published guidelines in a review on bacterial meningitis therapy, Michael Radetsky noted that [R]ecommended criteria, even if provisional, may inadvertently become invested with an independent power to force submission and prohibit deviation. The danger is that sensitivity to individual responsiveness and variability will be lost.[2] Guidelines are useful tools in pediatrics and should continue to be used to direct IV antibiotic durations for bacterial infections in children. However, the emphasis on fixed durations of IV antibiotics might not always serve the best interest of the patient. When guidelines are lacking or contradictory, patient factors should also be considered.
Acknowledgements
The authors thank Ellen R. Wald, MD, and Kenneth B. Roberts, MD, for their thoughtful and valuable additions to this review.
Disclosure: Nothing to report.
Rationally defining the appropriate duration of intravenous (IV) antibiotics for children with bacterial infections is challenging. For example, how long should a 2‐week‐old infant with a urinary tract infection (UTI) caused by Escherichia coli (E coli) be treated intravenously if the infant has responded to treatment and is back to baseline within 1 to 2 days? What if the blood culture was also positive for E coli? What are the risks and benefits of continuing IV antibiotics?
Such questions are common for pediatric hospitalists. Bacterial infections remain a relatively frequent cause of pediatric hospitalization, especially in neonates where 5 of the top 10 causes of hospitalizations are related to bacterial infections.[1] For some conditions, children remain hospitalized after clinical improvement simply for ongoing provision of IV antibiotics. Alternatively, some children are discharged home with a peripherally inserted central catheter (PICC) to complete an IV course.
The decision regarding the duration of IV antibiotics varies according to the condition for which the antibiotic is prescribed and often by practitioner or hospital. Many recommendations are numerically based (eg, 10 days for group B Streptococcus [GBS] bacteremia, 21 days for E coli meningitis), without taking into account patient‐level factors such as initial severity or response to therapy. These concrete recommendations may in fact be preferred by some practitioners, as suggested by a former chairman of the Committee on Infectious Disease for the American Academy of Pediatrics (AAP): The Red Book is designed for people who make decisions. It cannot waffle on an issue. It has to make a positive recommendation even if the data are incomplete.[2] A potential downside of this mentality, however, is that some practitioners may then feel obligated to follow these recommendations despite the lack of supportive evidence.
EXTENDING IV ANTIBIOTICS BEYOND CLINICAL RECOVERY
What is the rationale for continuing IV antibiotics in infants whose symptoms have completely resolved? Several factors likely drive these decisions: prevention of recurrences, concerns about bioavailability of enteral antibiotics and patient compliance, adherence to expert recommendations/guidelines, and perhaps a general sense that more is betterthat serious infections and/or their sequelae require more aggressive treatments.
Recurrence of a potentially life‐threatening infection is an understandable concern. Even when symptoms have resolved and there is documented clearance of the infection, such clearance does not necessarily signify that the body has rid itself of the pathogen completely. Some infections are deep seated and may warrant continuing treatment despite apparent recovery. To some, the risks of prolonging IV therapy may seem inconsequential when juxtaposed to a potentially devastating recurrence. However, in many conditions, recurrences may be related to host issues or ongoing exposures rather than inadequate treatment of the original infection. Recurrent UTIs, for example, are more likely in infants with urologic abnormalities,[3] and recurrent GBS bacteremia has been associated with GBS colonization of maternal breast milk and/or maternal mastitis.[4, 5, 6, 7] Although it is tempting to extend IV courses to prevent recurrences, it is not clear that the benefits of such an approach outweigh the risks.
Concerns over enteral absorption and bioavailability are also understandable, especially in young infants. The superior efficacy of IV over oral antibiotics in general is well accepted for many pediatric conditions, and in some cases (eg, septic shock) it would be unethical to perform a head‐to‐head trial. However, the lack of any published trials (to our knowledge) in pediatrics confirming the superiority of IV antibiotics suggests that oral antibiotic absorption is sufficient for many infections. Even in neonates, several studies have demonstrated that therapeutic serum levels are easily reached with oral dosing of amoxicillin in term and preterm neonates.[8, 9]
For the remainder of this review, the published recommendations and available evidence behind the duration of IV therapy are summarized for 4 bacterial infections in children in which IV antibiotic therapy often continues after clinical recovery: meningitis, bacteremia, UTI, and acute osteomyelitis. We conclude by proposing additional considerations for IV antibiotic durations, especially in situations where guidelines and/or evidence are either nonexistent, dated, conflicting, or contrary to evidence from published studies.
BACTERIAL MENINGITIS
The Infectious Disease Society of America and the British National Institute for Clinical Evidence have both published guidelines with pediatric recommendations for duration of therapy in bacterial meningitis,[10, 11] though the recommendations differ somewhat for 3 of the 4 most common pathogens, and are not always concordant with evidence from randomized controlled trials (Table 1).[12, 13, 14]
Pathogen | IDSA | NICE | Minimum Range Achieving Equivalent Outcomes in Recent Randomized Trials |
---|---|---|---|
| |||
Group B Streptococcus | 1421 days | 14 days | None available |
Neisseria meningititis | 7 days | 7 days | 15 days[12, 13, 14] |
Haemophilus influenzae type b | 7 days | 10 days | 45 days[12, 13] |
Streptococcus pneumoniae | 1014 days | 14 days | 45 days[12, 13] |
A recent meta‐analysis on duration of therapy in meningitis included 5 open‐label trials of ceftriaxone for bacterial meningitis in children.[12] These trials included the 3 most common pathogens and were categorized as short‐course (47 days, n=196 patients) and long‐course (714 days, n=187 patients) therapy. There was no significant difference in clinical success or long‐term neurological complications between groups. Subsequently, a multicountry trial enrolled over 1000 children 2 months to 12 years of age with meningitis caused by Haemophilus influenzae type b, Streptococcus pneumonia, or Neisseria meningititis who were stable after 5 days of IV ceftriaxone therapy and randomized them to receive placebo or an additional 5 days of ceftriaxone.[13] Patients with persistence of seizures, bacteremia, abscess or distant infections, or who were judged to be deteriorating or still severely ill at the 5‐day point were excluded (4.7% of the children who were recruited on day 0). There were no significant differences in bacteriologic failures, clinical failures, or clinical sequelae in survivors. The authors concluded that ceftriaxone can be discontinued in children with bacterial meningitis who are clinically stable after 5 days of IV therapy. Further trials in developed countries are needed.
BACTEREMIA
Because of routine vaccination against H influenzae type b and S pneumoniae, bacteremia beyond the first few months of life in otherwise healthy children is now rare.[15] Even in infants too young to benefit directly from vaccination, the epidemiology of bacteremia has changed considerably over the last few decades, with E coli and GBS constituting the majority (65%77%) of cases.[16, 17] We will limit this review on bacteremia to these 2 organisms in young infants.
Most cases of E coli bacteremia are associated with UTI (91%98%),[16, 17] and most bacteremic UTIs (88%92%) are caused by E coli.[18, 19, 20, 21] There are no official recommendations for the duration of treatment of bacteremic UTI, and only a limited amount of evidence can be gleaned from existing studies. In a trial of oral cefixime for infants aged 1 to 24 months with UTI, all 13 infants with bacteremia fared well whether they received oral cefixime only or IV cefotaxime for 3 days followed by oral cefixime.[18] In a study on length of IV antibiotic therapy in over 12,000 infants <6 months old with UTI, the presence of bacteremia predicted longer IV treatment length (bacteremia was present in 0.5% of the short IV group vs 0.8% of the long IV group, P=0.02) but did not predict treatment failure, defined as readmission within 30 days.[3] In a multicenter investigation of 229 infants <3 months old with bacteremic UTI, the duration of parenteral antibiotics was extremely variable (range, 117 days) and was not associated with treatment failure, defined as recurrent UTI caused by the same organism within 30 days (mean duration 7.8 days in the treatment‐failure group vs 7.7 days in the no‐failure group, P=0.99).[21] In summary, there is no evidence to support a prolonged course (ie, >35 days) of IV antibiotics for bacteremic UTI.
For bacteremia caused by GBS, although the Red Book Committee on Infectious Disease recommends 10 days of IV antibiotics,[22] to our knowledge there are no experimental or observational investigations to support this recommendation. Although available studies suggest that IV courses of at least 10 days are generally provided,[7, 23] no studies have compared outcomes of infants treated with short versus long courses. However, in a study that included 29 full‐term neonates with GBS bacteremia, all 29 had responded initially to 48 hours of intravenous antibiotics (defined as being asymptomatic and fed enterally), and were then treated successfully with high‐dose oral amoxicillin for the remainder of the course, with no recurrences.[8] Although recurrences are estimated to occur in 0.5% to 3% of babies treated for GBS infections, many recurrences are associated with exposure factors such as GBS colonization of the breast milk.[4, 5, 6, 7] In summary, although 10 or more days of IV antibiotic therapy remains a common published recommendation, there is no supportive evidence. More research is needed to assess whether shorter IV courses are safe.
UTI
Most UTIs can be treated with oral antibiotics.[24] In its practice parameter on febrile UTIs in infants 2 months to 2 years of age, the AAP recommends oral antibiotics for well‐appearing children.[25] This recommendation is supported by a recent Cochrane review on the topic,[26] and at least 3 additional trials that have demonstrated that long IV courses do not yield better outcomes than shorter IV courses or oral only courses.[27, 28, 29]
However, all of these trials exclude infants <1 month old, and there are no published recommendations for the <2‐month‐old age group. The study by Brady et al. on >12,000 infants <6 months old with UTI demonstrated no significant differences in UTI readmission rates between infants who were given 4 days of IV antibiotics versus those who were given <4 days.[3] There were 3,383 infants <30 days old in this study, and about one‐third of these babies received a short IV course. Failure rates were nearly identical in each group (2.3% in short course vs 2.4% in long course) even after risk adjustment (personal communication with Patrick Brady, MD, on February 7, 2014). Magin et al. describe 172 neonates (median age 19 days) with UTI who were treated intravenously for a median duration of 4 days (interquartile range, 36 days) and did not experience treatment failures or relapses.[30]
In summary, most cases of UTI can be managed with oral antibiotics. Uncertainty remains over the optimal approach for infants <1 to 2 months old, an age range not considered in current published guidelines. Current evidence suggests that IV treatment for 3 to 4 days followed by oral therapy may be sufficient treatment in this age group.
ACUTE OSTEOMYELITIS
Given the excellent blood supply to rapidly growing tissues in children, shorter durations of IV therapy have been studied with increasing frequency. A 2002 systematic review included 12 prospective cohort studies with at least 6 months of follow‐up.[31] Studies were stratified into 7 days or >7 days IV therapy, and there were no differences in cure rates. Subsequently, a large Finnish trial reported on 131 children who received an initial short IV course (24 days) followed by 20 versus 30 total days of therapy with very low treatment failure rates.[32]
The largest study from the United States to date analyzed nearly 2000 cases of osteomyelitis from 29 hospitals.[33] This study defined a prolonged IV course by placement of a central venous catheter. The rates of prolonged IV therapy varied significantly across hospitals, ranging from 10% to 95% of patients, without detectable differences in outcomes. Furthermore, the readmission rate for catheter related complications (3%) was nearly as high as the overall treatment failure rate (4%5%). Recently, Arnold et al. reported 8 years' experience with a management algorithm to guide the transition to oral antibiotics in pediatric osteoarticular infections in a patient specific manner.[34] This study included 194 patients (154 uncomplicated and 40 complicated cases), all with culture‐proven disease. Transition to oral antibiotics occurred based on resolution of fever and pain, improved function of the affected region, and a C‐reactive protein level of <3 mg/dL, and occurred at an average of 10 days into the treatment course. These authors also provided extensive information about complications to demonstrate that the proposed strategy can be used with a wide range of patients and pathogens. There was a single microbiologic treatment failure after oral step‐down therapy in a complicated osteoarticular infection, with a retained bony fragment. This study represents a successful example of a patient‐centered approach to IV antibiotic duration.
A PATIENT‐CENTERED APPROACH
Returning to the example above of the 2‐week‐old with UTI (with or without bacteremia), there are no published guidelines and only limited available evidence to help guide the duration of IV antibiotics in this case. When standards of care (eg, from published guidelines, review articles, textbooks, or local expert guidance) are nonexistent, conflicting, dated, or contrary to existing evidence, patient‐level factors can be incorporated into the decision‐making process (Table 2). In these cases, tailoring the IV antibiotic course to the individual's response (referred to in 1 review as the ultimate bioassay of the therapy[2]), while also weighing risks and benefits of ongoing therapy, is a logical approach.
Consideration | Description |
---|---|
| |
Severity of initial infection | If concern of recurrence is the justification for a longer IV course, then a more prolonged course might be considered for a more severe initial presentation (eg, septic shock, multisystem organ failure, intensive care unit admission). |
Response to therapy | Continued IV antibiotics might be warranted in patients who are still symptomatic (eg, fever, vomiting). Inflammatory markers have been used to guide therapy in osteomyelitis.[34] |
Patient compliance | If a child does not tolerate oral antibiotics or there are concerns about family adherence, a longer IV course may be considered. |
Family preferences | Shared decision making can be employed, especially when there is no clear evidence supporting a specific duration. |
Assessment of harms of ongoing hospitalization and/or prolonged IV therapy | See Table 3 |
SEVERITY OF INITIAL INFECTION AND RESPONSE TO THERAPY
The severity of the initial infection, whether in terms of presentation or clinical recovery, can factor into the duration of therapy. Provision of a longer IV course to prevent (albeit theoretically) a recurrence makes more logical sense in an infant with GBS bacteremia who was ill enough to warrant intensive care unit admission than in an infant whose only symptom was a fever. Similarly, most practitioners would be reluctant to stop IV antibiotics and discharge a patient with a bacterial infection who is persistently febrile or vomiting. Although the use of inflammatory markers and other clinical symptoms to guide therapy has been limited to osteomyelitis, this approach might be useful and should be studied in other conditions.
SHARED DECISION MAKING
Shared decision making can also be employed. Parents of sick, hospitalized children generally prefer to be involved in the decision‐making process.[35] For a parent who has concerns about their child's well‐being in the hospital, or has multiple other children at home, competing career obligations, and/or limited family support, the burden of ongoing hospitalization can be significant, and should be factored into decision making. Involving parents in medical decisions may lead to a reduction in utilization for some conditions.[36]
ASSESSMENT OF RISKS/COSTS
The risks and costs of pediatric hospitalization and prolonged IV antibiotics are well described in the literature and are summarized in Table 3. Although the benefits of prolonging IV antibiotics in a child who has recovered from an acute bacterial infection are largely theoretical, many of the risks are concrete and quantifiable. For example, a young infant being treated for a bacteremic UTI may run out of potential IV sites and need a PICC line to continue IV therapy, which according to a recent review of 2574 PICC lines has a 21% complication rate. This rate is even higher in children for whom the PICC line indication was provision of antibiotics (27%) and for infants <1 year of age (44%).[37] Moreover, this procedure often requires sedation or anesthesia for placement, which has both known and unknown risks, including concerns about subsequent adverse effects on development in young children.[38] Nosocomial exposure to seasonal viruses poses an additional risk to hospitalized children.[39]
Harm of Intravenous Antibiotic Therapy | Description or Example |
---|---|
| |
Complications from peripheral IV catheter | Leading source of pain and distress for hospitalized children.[44] |
Serious complications can occur following IV infiltrates.[45] | |
Complications from PICC line | Approximately 20% overall complication rate (44% in infants <1 year old).[37] |
Complications led to rehospitalization of 3% of children being treated with prolonged antibiotics for osteomyelitis.[33] | |
When thrombosis occurs (up to 9% risk in neonates[46]), 3 months of anticoagulation is recommended.[47] | |
Complications may arise from sedation/anesthesia necessary to place catheter. Anesthesia has been associated with adverse behavioral or developmental outcomes in children <4 years of age.[38] | |
Risk of nosocomial infection while hospitalized | An estimated 6% of hospital RSV infections are nosocomial, which are associated with a more prolonged LOS than hospitalizations for community‐acquired RSV.[39] |
Medication error | In 1 investigation, serious medication errors occurred in 22 per 1,000 patient‐days in a large children's hospital.[48] |
Emotional and financial burdens | Hospitalization can pose a significant strain on the child, parents, and siblings. |
Financial costs to healthcare system | In 2003, infection‐related hospitalizations in infants had an average cost of $4,000 (average LOS 3.5 days).[1] |
Harms associated with prolonged courses of antibiotics in general (IV or PO) | Antibiotic resistance, diarrhea (including Clostridium difficile), allergic reactions, increased costs.[49] |
These additional considerations for the duration of IV antibiotics are not evidence based and should not be used to justify an IV duration that differs dramatically from an accepted standard of care. These are merely considerations that incorporate clinical judgment and a comprehensive analysis of risks and benefits in situations where the available evidence is suboptimal. This approach can be adopted both as a framework for future research and directly in clinical practice.
CONCLUSION
In an era of increasing focus on overtreatment/waste,[40] patient safety,[41] and patient‐centered care,[42] the duration of IV antibiotics for common bacterial infections is a prime target for improving pediatric healthcare value. As emphasized by Michael Porter recently in The New England Journal of Medicine, value should always be defined around the customer.[43] A high‐value approach to IV antibiotic duration incorporates a rigorous assessment of risks and benefits that focuses on best evidence and patient‐level factors.
In discussing published guidelines in a review on bacterial meningitis therapy, Michael Radetsky noted that [R]ecommended criteria, even if provisional, may inadvertently become invested with an independent power to force submission and prohibit deviation. The danger is that sensitivity to individual responsiveness and variability will be lost.[2] Guidelines are useful tools in pediatrics and should continue to be used to direct IV antibiotic durations for bacterial infections in children. However, the emphasis on fixed durations of IV antibiotics might not always serve the best interest of the patient. When guidelines are lacking or contradictory, patient factors should also be considered.
Acknowledgements
The authors thank Ellen R. Wald, MD, and Kenneth B. Roberts, MD, for their thoughtful and valuable additions to this review.
Disclosure: Nothing to report.
- Infectious disease hospitalizations among infants in the United States. Pediatrics. 2008;121(2):244–252. , , , , .
- Duration of treatment in bacterial meningitis: a historical inquiry. Pediatr Infect Dis J. 1990;9(1):2–9. .
- Length of intravenous antibiotic therapy and treatment failure in infants with urinary tract infections. Pediatrics. 2010;126(2):196–203. , , .
- Group B streptococci in milk and late neonatal infections: an analysis of cases in the literature. Arch Dis Child Fetal Neonatal Ed. 2014;99(1):F41–F47. , , , et al.
- Recurrent late‐onset group B Streptococcus sepsis in a preterm infant acquired by expressed breastmilk transmission: a case report. Breastfeed Med. 2013;8(1):134–136. , , .
- Late‐onset and recurrent neonatal Group B streptococcal disease associated with breast‐milk transmission. Pediatr Dev Pathol. 2003;6(3):251–256. , , , , , .
- A 5‐year review of recurrent group B streptococcal disease: lessons from twin infants. Clin Infect Dis. 2000;30(2):282–287. , , , , .
- Therapeutic amoxicillin levels achieved with oral administration in term neonates. Eur J Clin Pharmacol. 2007;63(7):657–662. , , , et al.
- Population pharmacokinetics and dosing of amoxicillin in (pre)term neonates. Ther Drug Monit. 2006;28(2):226–231. , , , , , .
- Practice guidelines for the management of bacterial meningitis. Clin Infect Dis. 2004;39(9):1267–1284. , , , et al.
- Management of bacterial meningitis and meningococcal septicaemia in children and young people: summary of NICE guidance. BMJ. 2010;340:c3209. , , , , , .
- Short versus long duration of antibiotic therapy for bacterial meningitis: a meta‐analysis of randomised controlled trials in children. Arch Dis Child. 2009;94(8):607–614. , , , , .
- 5 versus 10 days of treatment with ceftriaxone for bacterial meningitis in children: a double‐blind randomised equivalence study. Lancet. 2011;377(9780):1837–1845. , , , et al.
- Ceftriaxone as effective as long‐acting chloramphenicol in short‐course treatment of meningococcal meningitis during epidemics: a randomised non‐inferiority study. Lancet. 2005;366(9482):308–313. , , , et al.
- Changing epidemiology of outpatient bacteremia in 3‐ to 36‐month‐old children after the introduction of the heptavalent‐conjugated pneumococcal vaccine. Pediatr Infect Dis J. 2006;25(4):293–300. , , , et al.
- Epidemiology of bacteremia in febrile infants in the United States. Pediatrics. 2013;132(6):990–996. , , , et al.
- Changing epidemiology of bacteremia in infants aged 1 week to 3 months. Pediatrics. 2012;129(3):e590–e596. , , .
- Oral versus initial intravenous therapy for urinary tract infections in young febrile children. Pediatrics. 1999;104(1 pt 1):79–86. , , , et al.
- Bacteremic urinary tract infection in children. Pediatr Infect Dis J. 2000;19(7):630–634. , , , , .
- Urine testing and urinary tract infections in febrile infants seen in office settings: the Pediatric Research in Office Settings' Febrile Infant Study. Arch Pediatr Adolesc Med. 2002;156(1):44–54. , , , , , .
- 2014; Vancouver BC, Canada. , , , , , . Management of bacteremic urinary tract infections in infants less than 3 months of age. Abstract presented at: Pediatric Academic Societies Annual Meeting; May 5,
- Red Book: 2009 Report of the Committee on Infectious Diseases. 28th ed. Elk Grove Village, IL: American Academy of Pediatrics; 2012. , , , .
- Recurrent group B streptococcal bacteremia. Clin Pediatr (Phila). 2012;51(9):884–887. , .
- Antibiotics for treating lower urinary tract infection in children. Cochrane Database Syst Rev. 2012;8:CD006857. , , , .
- Urinary tract infection: clinical practice guideline for the diagnosis and management of the initial UTI in febrile infants and children 2 to 24 months. Pediatrics. 2011;128(3):595–610. .
- Antibiotics for acute pyelonephritis in children. Cochrane Database Syst Rev. 2007(4):CD003772. , , .
- Randomized trial of oral versus sequential IV/oral antibiotic for acute pyelonephritis in children. Pediatrics. 2012;129(2):e269–e275. , , , et al.
- Prospective, randomized trial comparing short and long intravenous antibiotic treatment of acute pyelonephritis in children: dimercaptosuccinic acid scintigraphic evaluation at 9 months. Pediatrics. 2008;121(3):e553–e560. , , , et al.
- Randomised trial of oral versus sequential intravenous/oral cephalosporins in children with pyelonephritis. Eur J Pediatr. 2008;167(9):1037–1047. , , , et al.
- Efficacy of short‐term intravenous antibiotic in neonates with urinary tract infection. Pediatr Emerg Care. 2007;23(2):83–86. , , , , .
- Shorter courses of parenteral antibiotic therapy do not appear to influence response rates for children with acute hematogenous osteomyelitis: a systematic review. BMC Infect Dis. 2002;2:16. , , , , , .
- Short‐ versus long‐term antimicrobial treatment for acute hematogenous osteomyelitis of childhood: prospective, randomized trial on 131 culture‐positive cases. Pediatr Infect Dis J. 2010;29(12):1123–1128. , , , .
- Prolonged intravenous therapy versus early transition to oral antimicrobial therapy for acute osteomyelitis in children. Pediatrics. 2009;123(2):636–642. , , , , , .
- Acute bacterial osteoarticular infections: eight‐year analysis of C‐reactive protein for oral step‐down therapy. Pediatrics. 2012;130(4):e821–e828. , , , et al.
- Parental decision‐making preferences in the pediatric intensive care unit. Crit Care Med. 2012;40(10):2876–2882. , , , , , .
- An assessment of the shared‐decision model in parents of children with acute otitis media. Pediatrics. 2005;116(6):1267–1275. , , , , .
- Risk factors for peripherally inserted central venous catheter complications in children. JAMA Pediatr. 2013;167(5):429–435. , , , , .
- Current clinical evidence on the effect of general anesthesia on neurodevelopment in children: an updated systematic review with meta‐regression. PLoS One. 2014;9(1):e85760. , , .
- Nosocomial respiratory syncytial virus infection in Canadian pediatric hospitals: a Pediatric Investigators Collaborative Network on Infections in Canada Study. Pediatrics. 1997;100(6):943–946. , , , et al.
- Eliminating waste in US health care. JAMA. 2012;307(14):1513–1516. , .
- Safely doing less: a missing component of the patient safety dialogue. Pediatrics. 2011;128(6):e1596–e1597. , , .
- Committee On Hospital Care and Institute For Patient‐ and Family‐Centered Care. Patient‐ and family‐centered care and the pediatrician's role. Pediatrics. 2012;129(2):394–404.
- What is value in health care? N Engl J Med. 2010;363(26):2477–2481. .
- Prevalence and source of pain in pediatric inpatients. Pain. 1996;68(1):25–31. , , , , .
- Acute compartment syndrome of the upper extremity in children: diagnosis, management, and outcomes. J Child Orthop. 2013;7(3):225–233. , , , .
- Neonatal central venous catheter thrombosis: diagnosis, management and outcome. Blood Coagul Fibrinolysis. 2014;25(2):97–106. , , , , .
- Antithrombotic therapy in neonates and children: antithrombotic therapy and prevention of thrombosis, 9th ed: American C ollege of Chest Physicians Evidence‐Based Clinical Practice Guidelines. Chest. 2012;141(2 suppl):e737S–801S. , , , et al.
- Effect of computer order entry on prevention of serious medication errors in hospitalized children. Pediatrics. 2008;121(3):e421–e427. , , , et al.
- Duration and cessation of antimicrobial treatment. J Hosp Med. 2012;7(suppl 1):S22–S33.
- Infectious disease hospitalizations among infants in the United States. Pediatrics. 2008;121(2):244–252. , , , , .
- Duration of treatment in bacterial meningitis: a historical inquiry. Pediatr Infect Dis J. 1990;9(1):2–9. .
- Length of intravenous antibiotic therapy and treatment failure in infants with urinary tract infections. Pediatrics. 2010;126(2):196–203. , , .
- Group B streptococci in milk and late neonatal infections: an analysis of cases in the literature. Arch Dis Child Fetal Neonatal Ed. 2014;99(1):F41–F47. , , , et al.
- Recurrent late‐onset group B Streptococcus sepsis in a preterm infant acquired by expressed breastmilk transmission: a case report. Breastfeed Med. 2013;8(1):134–136. , , .
- Late‐onset and recurrent neonatal Group B streptococcal disease associated with breast‐milk transmission. Pediatr Dev Pathol. 2003;6(3):251–256. , , , , , .
- A 5‐year review of recurrent group B streptococcal disease: lessons from twin infants. Clin Infect Dis. 2000;30(2):282–287. , , , , .
- Therapeutic amoxicillin levels achieved with oral administration in term neonates. Eur J Clin Pharmacol. 2007;63(7):657–662. , , , et al.
- Population pharmacokinetics and dosing of amoxicillin in (pre)term neonates. Ther Drug Monit. 2006;28(2):226–231. , , , , , .
- Practice guidelines for the management of bacterial meningitis. Clin Infect Dis. 2004;39(9):1267–1284. , , , et al.
- Management of bacterial meningitis and meningococcal septicaemia in children and young people: summary of NICE guidance. BMJ. 2010;340:c3209. , , , , , .
- Short versus long duration of antibiotic therapy for bacterial meningitis: a meta‐analysis of randomised controlled trials in children. Arch Dis Child. 2009;94(8):607–614. , , , , .
- 5 versus 10 days of treatment with ceftriaxone for bacterial meningitis in children: a double‐blind randomised equivalence study. Lancet. 2011;377(9780):1837–1845. , , , et al.
- Ceftriaxone as effective as long‐acting chloramphenicol in short‐course treatment of meningococcal meningitis during epidemics: a randomised non‐inferiority study. Lancet. 2005;366(9482):308–313. , , , et al.
- Changing epidemiology of outpatient bacteremia in 3‐ to 36‐month‐old children after the introduction of the heptavalent‐conjugated pneumococcal vaccine. Pediatr Infect Dis J. 2006;25(4):293–300. , , , et al.
- Epidemiology of bacteremia in febrile infants in the United States. Pediatrics. 2013;132(6):990–996. , , , et al.
- Changing epidemiology of bacteremia in infants aged 1 week to 3 months. Pediatrics. 2012;129(3):e590–e596. , , .
- Oral versus initial intravenous therapy for urinary tract infections in young febrile children. Pediatrics. 1999;104(1 pt 1):79–86. , , , et al.
- Bacteremic urinary tract infection in children. Pediatr Infect Dis J. 2000;19(7):630–634. , , , , .
- Urine testing and urinary tract infections in febrile infants seen in office settings: the Pediatric Research in Office Settings' Febrile Infant Study. Arch Pediatr Adolesc Med. 2002;156(1):44–54. , , , , , .
- 2014; Vancouver BC, Canada. , , , , , . Management of bacteremic urinary tract infections in infants less than 3 months of age. Abstract presented at: Pediatric Academic Societies Annual Meeting; May 5,
- Red Book: 2009 Report of the Committee on Infectious Diseases. 28th ed. Elk Grove Village, IL: American Academy of Pediatrics; 2012. , , , .
- Recurrent group B streptococcal bacteremia. Clin Pediatr (Phila). 2012;51(9):884–887. , .
- Antibiotics for treating lower urinary tract infection in children. Cochrane Database Syst Rev. 2012;8:CD006857. , , , .
- Urinary tract infection: clinical practice guideline for the diagnosis and management of the initial UTI in febrile infants and children 2 to 24 months. Pediatrics. 2011;128(3):595–610. .
- Antibiotics for acute pyelonephritis in children. Cochrane Database Syst Rev. 2007(4):CD003772. , , .
- Randomized trial of oral versus sequential IV/oral antibiotic for acute pyelonephritis in children. Pediatrics. 2012;129(2):e269–e275. , , , et al.
- Prospective, randomized trial comparing short and long intravenous antibiotic treatment of acute pyelonephritis in children: dimercaptosuccinic acid scintigraphic evaluation at 9 months. Pediatrics. 2008;121(3):e553–e560. , , , et al.
- Randomised trial of oral versus sequential intravenous/oral cephalosporins in children with pyelonephritis. Eur J Pediatr. 2008;167(9):1037–1047. , , , et al.
- Efficacy of short‐term intravenous antibiotic in neonates with urinary tract infection. Pediatr Emerg Care. 2007;23(2):83–86. , , , , .
- Shorter courses of parenteral antibiotic therapy do not appear to influence response rates for children with acute hematogenous osteomyelitis: a systematic review. BMC Infect Dis. 2002;2:16. , , , , , .
- Short‐ versus long‐term antimicrobial treatment for acute hematogenous osteomyelitis of childhood: prospective, randomized trial on 131 culture‐positive cases. Pediatr Infect Dis J. 2010;29(12):1123–1128. , , , .
- Prolonged intravenous therapy versus early transition to oral antimicrobial therapy for acute osteomyelitis in children. Pediatrics. 2009;123(2):636–642. , , , , , .
- Acute bacterial osteoarticular infections: eight‐year analysis of C‐reactive protein for oral step‐down therapy. Pediatrics. 2012;130(4):e821–e828. , , , et al.
- Parental decision‐making preferences in the pediatric intensive care unit. Crit Care Med. 2012;40(10):2876–2882. , , , , , .
- An assessment of the shared‐decision model in parents of children with acute otitis media. Pediatrics. 2005;116(6):1267–1275. , , , , .
- Risk factors for peripherally inserted central venous catheter complications in children. JAMA Pediatr. 2013;167(5):429–435. , , , , .
- Current clinical evidence on the effect of general anesthesia on neurodevelopment in children: an updated systematic review with meta‐regression. PLoS One. 2014;9(1):e85760. , , .
- Nosocomial respiratory syncytial virus infection in Canadian pediatric hospitals: a Pediatric Investigators Collaborative Network on Infections in Canada Study. Pediatrics. 1997;100(6):943–946. , , , et al.
- Eliminating waste in US health care. JAMA. 2012;307(14):1513–1516. , .
- Safely doing less: a missing component of the patient safety dialogue. Pediatrics. 2011;128(6):e1596–e1597. , , .
- Committee On Hospital Care and Institute For Patient‐ and Family‐Centered Care. Patient‐ and family‐centered care and the pediatrician's role. Pediatrics. 2012;129(2):394–404.
- What is value in health care? N Engl J Med. 2010;363(26):2477–2481. .
- Prevalence and source of pain in pediatric inpatients. Pain. 1996;68(1):25–31. , , , , .
- Acute compartment syndrome of the upper extremity in children: diagnosis, management, and outcomes. J Child Orthop. 2013;7(3):225–233. , , , .
- Neonatal central venous catheter thrombosis: diagnosis, management and outcome. Blood Coagul Fibrinolysis. 2014;25(2):97–106. , , , , .
- Antithrombotic therapy in neonates and children: antithrombotic therapy and prevention of thrombosis, 9th ed: American C ollege of Chest Physicians Evidence‐Based Clinical Practice Guidelines. Chest. 2012;141(2 suppl):e737S–801S. , , , et al.
- Effect of computer order entry on prevention of serious medication errors in hospitalized children. Pediatrics. 2008;121(3):e421–e427. , , , et al.
- Duration and cessation of antimicrobial treatment. J Hosp Med. 2012;7(suppl 1):S22–S33.
Choosing Wisely in Pediatric Medicine
Overuse in medicine is a significant and under‐recognized problem. Don Berwick estimated that waste accounts for at least 20% of healthcare expenditures in the United States, with overtreatment as one of the largest categories.[1] A commentary by Schroeder et al. challenged pediatricians to incorporate this knowledge into our own patient safety and quality movement.[2] Recently published data suggest that we are far from achieving the patient safety goals set forth in the Institute of Medicine's landmark To Err is Human[3] report, despite more than a decade of national, local, and regional efforts.[4] One way to reduce waste and improve patient safety is to eliminate practices of unproven benefit. Therapies or tests that may initially seem promising are often proven to be not only unhelpful but actually harmful. The recommendation of the US Preventive Services Task Force against routine screening for prostate specific antigen is an example of how a common test initially thought of as lifesaving actually increases harm.[5]
The American Board of Internal Medicine Foundation (ABIM‐F) recently announced the Choosing Wisely campaign. Through this campaign the Foundation encourages physicians, patients and other healthcare stakeholders to think and talk about medical tests and procedures that may be unnecessary.[6] The primary output of this challenge is the development of a list of 5 tests and or therapies that physicians and patients should question. The ABIM‐F approached different medical societies to develop these lists within their own specialties. The Society of Hospital Medicine (SHM) joined the Choosing Wisely campaign in April 2012, and agreed to develop a list of 5 therapies and tests for adult hospital medicine and pediatric hospital medicine. Here we present the contribution of the pediatric workgroup detailing the methodology and process for developing the list, as well as summarizing the evidence supporting each recommendation.
METHODS
In the spring of 2012, the pediatric committee of the SHM convened a workgroup of pediatric hospitalists to develop a top 5 list for the field. This workgroup was composed of experienced pediatric hospitalists representing diverse geographic locations of the United States and a mix of academic and nonacademic practice settings. The group, consisting of 4 women and 9 men, began by proposing candidate recommendations after discussion with colleagues at their different practice sites. The group was charged to maintain a focus on overuse practices that had a strong basis in evidence, were frequently encountered at their practice sites, and achieved significant consensus among their colleagues. Figure 1 shows the process map describing the method for the development of the pediatric recommendations. All workgroup participants were queried as to conflict of interest relevant to this work and none were identified.
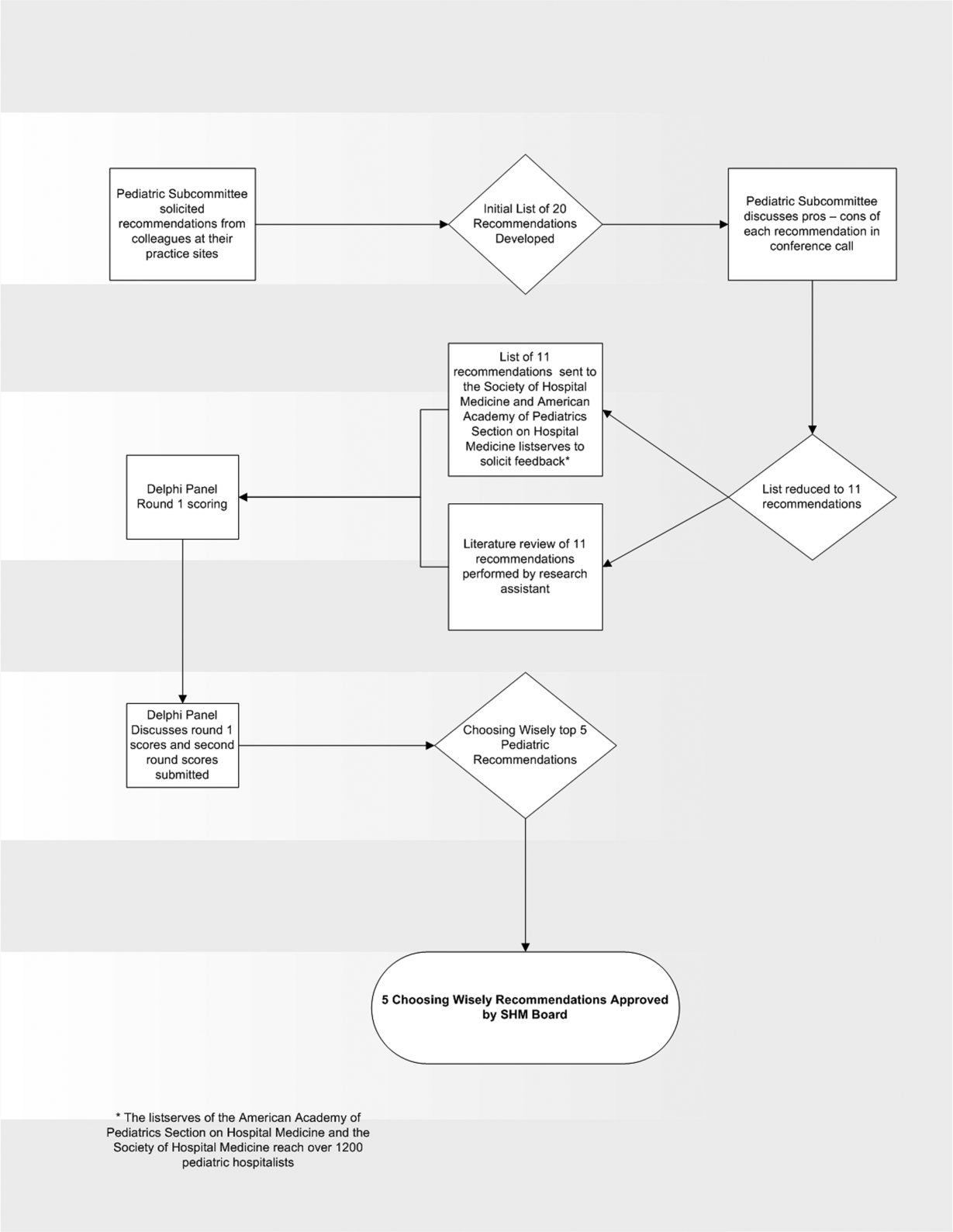
Literature Review
After the generation of the initial top 20 list, 2 reviewers conducted independent literature searches in PubMed, MEDLINE, and the Cochrane Library on the proposed topics. The reviewers also conducted generic Internet searches. Key search terms included pediatric asthma, bronchiolitis, chest radiograph, systemic corticosteroids, gastroesophageal reflux disease (GERD), infant, child, acid suppression therapy, continuous pulse oximetry, pneumonia, gastroenteritis, viral testing, blood culture, and soft tissue infections. To ensure that the reviewers included all studies relevant to the searches, they utilized broad terms. The search included all literature published through 2012, and nonEnglish language publications were included in the search. Studies selected and included in the review were based upon common criteria including whether the article discussed an evaluation of efficacy and/or utility of treatment, included a pediatric population in the guidelines or study, reviewed the harm associated with the administration of a particular test or treatment, and explored the cost associated with the test or treatment.
The Delphi Panel
Members of the workgroup formed a Delphi panel except for 1 member (R.Q.) who served as the nonvoting moderator. The members of the Delphi panel considered the results of the literature search for each recommendation along with the collated feedback from hospitalist listserves as described in Figure 1. Each panel member received a voting instrument with the candidate tests and treatments for the first round of Delphi voting. The panel utilized a modified Delphi method or the RAND Corporation (RAND)/University of California at Los Angeles (UCLA) appropriateness method as described in previous publications of quality indicator development in pediatrics.[7] Each panelist scored the candidate tests and treatments and forwarded the scores to the moderator. Subsequently, all the members of the Delphi panel met through a conference call to carry out the second round of voting. The deidentified collated results of the first round of Delphi voting were made available and discussed during the call. The moderator collated the final results, and the final 5 recommendations were those that had the highest score after the second round of Delphi voting.
Volume and Costs
During deliberations, the committee took into account the prevalence and cost rankings of our most common pediatric inpatient diagnoses. This was done using the Agency for Healthcare Research and Quality's (AHRQ) Healthcare Utilization Project (HCUP), specifically, the Kids' Inpatient Database (KID). HCUP includes the largest collection of longitudinal hospital care data in the United States, encompassing all‐payer discharge‐level information. We excluded normal newborn hospitalizations, and looked at the top 10 acute inpatient diagnoses in terms of both volume and aggregate costs.
RESULTS
The initial list of 20 candidate tests and treatments as well as the refined list of 11 recommendations can be found as electronic supplements to this publication (see Supporting Table 1 and Supporting Table 2 in the online version of this article). The format and language of the list of 11 recommendations were chosen to mesh with that typically used in the ABIM‐F Choosing Wisely campaign. During the Delphi panel, there was strong group consensus about combining items 1 and 2 (chest radiographs in asthma and bronchiolitis) into a single recommendation.
Do not order chest radiographs in children with asthma or bronchiolitis. |
Do not use bronchodilators in children with bronchiolitis. |
Do not use systemic corticosteroids in children under 2 years of age with a lower respiratory tract infection. |
Do not treat gastroesophageal reflux in infants routinely with acid suppression therapy. |
Do not use continuous pulse oximetry routinely in children with acute respiratory illness unless they are on supplemental oxygen. |
The top 5 recommendations based on the result of the second round of Delphi scoring are shown in Table 1 and described below along with a detailed evidence summary.
Do not order chest radiographs in children with asthma or bronchiolitis.
The National Heart and Lung Institute's guidelines for the management of asthma, published in 1987, recommend against routinely obtaining chest radiographs in patients with asthma or asthma exacerbations.[8] Supporting this recommendation are several studies that show a low overall yield when obtaining chest radiographs for wheezing patients.[9, 10, 11] Most relevant, studies that evaluated the clinical utility of radiographs in patients with asthma have demonstrated that they influence clinical management in less than 2% of cases.[12] A quality improvement project aimed at decreasing the rate of chest radiographs obtained in patients with asthma demonstrated that close to 60% of patients admitted to the hospital had chest radiographs performed, and that significant overall reductions can be achieved (45.3%28.9%, P=0.0005) without impacting clinical outcomes negatively.[13]
Similarly, the Subcommittee on Diagnosis and Management of Bronchiolitis of the American Academy of Pediatrics recommends against routinely obtaining radiographs during the evaluation for bronchiolitis.[14] Studies assessing the utility of chest x‐rays in these children demonstrate an even lower incidence of abnormalities (0.75%) and indicate that, despite this low incidence, physicians are more likely to treat with antibiotics when radiographs are obtained.[15] There is also evidence that chest radiographs in patients with bronchiolitis are not useful in predicting severity of illness.[16] Furthermore, cost‐effective analyses have demonstrated that omitting chest radiographs in bronchiolitis is actually cost‐effective, without compromising diagnostic accuracy.[17] In a recently published national benchmarking inpatient collaborative, Ralston et al. demonstrated that the majority of patients admitted to the hospital with bronchiolitis have chest radiographs performed at a rate of 64% (interquartile range [IQR], 54%81%).[18]
In both bronchiolitis and asthma, the elimination of unnecessary radiographs has the potential to decrease costs, reduce radiation exposure, and minimize the overuse of antibiotics that often occurs secondary to false positive results.
Do not use bronchodilators in children with bronchiolitis.
Ralston showed that 70% (IQR, 59%83%) of admitted bronchiolitis patients received bronchodilators with an average of 7.9 doses per patient (IQR, 4.69.8). National guidelines for bronchiolitis suggest a very limited role of bronchodilators in patients with bronchiolitis.[14] The first meta‐analyses of studies related to the question of ‐agonist efficacy in bronchiolitis were published in the late 1990s, revealing minimal or no treatment effects.[19, 20] Since then, further research has solidified these findings, and fairly definitive statements can be made based on a recent comprehensive meta‐analysis.[21] The pooled data do not show any effect on hospitalization rates, hospital length of stay, or other inpatient outcomes in bronchiolitis. They do show a small change in clinical scores documented in the outpatient setting, though these scores have not correlated with any detectable difference in outcomes. Routine use of ‐agonists in the inpatient setting has no proven benefit, and given the large amount of consistent data, there is no compelling reason for further study of this therapy in the inpatient setting.
Epinephrine, a combined ‐ and ‐agonist, has been extensively evaluated in bronchiolitis as well. Like albuterol, epinephrine has been reported to have no effect on hospital length of stay in bronchiolitis.[22] The issue of admission rates after epinephrine is complicated by 1 very large study that combined epinephrine with dexamethasone and reported a decreased admission rate, though only at 7 days after therapy; however, this effect was nullified after adjustment for multiple comparisons.[23] When the end point is improvement of respiratory scores, epinephrine may perform better than albuterol in studies where they are directly compared; however, there is no evidence that repeated usage of epinephrine has any impact on any clinical outcome for inpatients.[24, 25]
Do not use systemic corticosteroids in children under 2 years of age with a lower respiratory tract infection
In their summary of evidence, the Subcommittee on Diagnosis and Management of Bronchiolitis of the American Academy of Pediatrics recommends against routinely using systemic corticosteroids for infants with bronchiolitis.[14] The previously reference bronchiolitis benchmarking study demonstrated that admitted patients received steroids at a rate of 21% (IQR, 14%26%). The poor efficacy of corticosteroids in children with bronchiolitis under 2 years of age is well demonstrated in the literature. A large, blinded, randomized, controlled study compared systemic oral corticosteroids to placebo in hospitalized children 10 months to 6 years of age with viral wheezing.[26] This study showed no benefit of corticosteroids over placebo in length of stay or parental report of symptoms 1 week later. In the study, a subanalysis of children with eczema and family history of asthma also demonstrated no benefit of systemic corticosteroids. Large systematic reviews further argue that there is no effect of corticosteroids on the likelihood of admission or length of stay in infants with bronchiolitis.[27, 28] One 4‐armed prospective study of children 6 weeks to 12 months of age found no efficacy of dexamethasone over placebo.[23] There was modest benefit of dexamethasone in conjunction with racemic epinephrine; however, this benefit disappeared after adjustment for multiple comparisons. Three smaller studies showing benefit of systemic corticosteroids, however, were highly problematic. They have included older children, were retrospective, or demonstrated inconsistent results.[29, 30] A smaller study showed benefit for children over 2 years of age, but none for children under 2 years of age.[31] Premature infants are at increased risk of asthma, which typically responds well to corticosteroids as these children get older. However, a retrospective study of premature infants under 2 years of age with bronchiolitis demonstrated no association between corticosteroid use and length of stay, even in the subset of premature infants responding to albuterol.[32]
Systemic corticosteroid use in children is not harmless. Children under 2 years of age are especially vulnerable to the decreased growth velocity seen as a side effect of systemic corticosteroids.[33] Corticosteroids may also negatively impact the course of infectious illness. For instance, in children hospitalized with pneumonia but not receiving ‐agonists (ie, patients who are unlikely to have asthma), length of stay is prolonged and readmission is higher in those who receive corticosteroids.[34]
Do not treat gastroesophageal reflux in infants routinely with acid suppression therapy.
From 2000 to 2005, the incidence of infants diagnosed with gastroeshopaheal reflux (GER) tripled (3.4%12.3%), and the use of proton pump inhibitors (PPIs) doubled (31.5%62.6%).[35] Patients diagnosed with GER and treated with antireflux medication incurred 1.8 times higher healthcare costs in 1 study compared to healthy controls.[36] Though common, the use of acid suppressive medications in infants lacks evidence for efficacy in the majority of the clinical scenarios in which they are prescribed.[37, 38] PPIs have failed to outperform placebo for typical infant reflux, which is generally developmental and not pathologic.[39, 40] Furthermore, prompted by findings in adults, multiple pediatric investigators have now catalogued the potential risks associated with acid blockade in children in multiple clinical settings. Specifically, increased risk of pneumonia has been documented in inpatients and outpatients, and increased risk of necrotizing enterocolitis and other serious infections have been documented in intensive care unit settings.[41] In the absence of data supporting efficacy and given the emerging data on risk, empiric acid suppression in infants with reflux is wasteful and potentially harmful.
Do not use continuous pulse oximetry routinely in children with acute respiratory illness unless they are on supplemental oxygen.
Pulse oximetry use has become widespread in the management of infants with bronchiolitis and likely accounts for the dramatic increase in bronchiolitis hospitalization rates in recent years.[14, 42, 43, 44, 45, 46, 47] Despite this increase in hospitalization rate, there was no change in mortality from bronchiolitis between 1979 and 1997.[48] The continuous monitoring of oxygen saturations in hospitalized infants with bronchiolitis may lead to overdiagnosis of hypoxemia and subsequent oxygen use that is of no apparent benefit to the child. Schroeder et al. demonstrated that 26% of a sample of infants hospitalized with bronchiolitis had a prolonged length of stay because of a perceived need for oxygen based on pulse oximetry readings.[43] Unger and Cunningham showed that the need for oxygen was the final determinant of length of stay in 58% of cases, and Cunningham and Murray suggested that using an oxygen saturation cutoff of 94% instead of 90% might increase the length of stay by 22 hours.[44, 49]
It has been previously shown that hypoxia is normative in infants. Healthy infants experience multiple episodes of SpO2 90% while sleeping.[50] This finding strengthens the notion that detection of low saturations in infants convalescing from bronchiolitis may simply reflect overdiagnosis. Among children with chronic severe asthma, who presumably have experienced episodes of hypoxia throughout childhood, there is no difference in school performance compared to healthy controls.[51]
The practice parameter on bronchiolitis from the American Academy of Pediatrics states: as the child's clinical course improves, continuous measurement of SpO2 is not routinely needed, which is a recommendation based on expert consensus.[14] There is at least one ongoing randomized trial comparing the use of continuous versus intermittent pulse oximetry in hospitalized infants with bronchiolitis who are weaned off oxygen (
DISCUSSION
Berwick and Hackbarth define overtreatment as: waste that comes from subjecting patients to care that, according to sound science and the patients' own preferences, cannot possibly help themcare rooted in outmoded habits, supply‐driven behaviors, and ignoring science.[1] With this project, we tried to capture common clinical sources of waste in the inpatient pediatric setting. This is an inherently difficult project because of the absence of solid evidence to inform every decision point in medicine. Although there is always room for improvement in our evidence base, our group intentionally gravitated to areas where the evidence was robust.
The primary strength of this work is the use of the RAND/UCLA appropriateness method or modified Delphi method. Several publications have validated this methodology as a sound strategy to assess quality indicators and issues related to overuse.[7, 53] To our knowledge, we are the first group to report the use of this methodology to develop a list such as the list reported here.
There were some challenges inherent to this project that can be considered limitations of the work. One perceived limitation of our list is the heavy concentration on respiratory diagnoses, especially bronchiolitis and asthma. We do not feel this is a genuine limitation, as the recommendations were partly driven by volume and costs as assessed by the KID database. Among the top 10 acute inpatient diagnoses in pediatrics, respiratory diagnoses are the most common, including bronchiolitis, pneumonia, and asthma. Pneumonia or bronchiolitis has been the most common medical diagnosis in inpatient pediatrics for the past decade, and both are always in the top 10 for costs as well.[54] Thus, the impact of decreasing overuse for these conditions will be highly significant from a simple volume standpoint.
The primary limitation of this work is the lack of implementation strategies. Although the Choosing Wisely campaign has plans for dissemination of the lists, compliance with the recommendations may be suboptimal. Although the development process followed an accepted methodology, shortcomings include the lack of wide, local, multidisciplinary (including parents or caretakers) consultation. Other barriers to compliance with these recommendations exist. Despite evidence that bronchiolitis is a benign self‐limited disease that does not respond to bronchodilators and steroids, the drive to identify and correct all abnormalities, such as wheezing or low oxygen saturation in a nontoxic infant with bronchiolitis, seems to trump the obligation to do no harm in daily practice.[55] This behavior may result from pressure by patients, families, nurses, or peers and is deeply embedded in our medical culture, where action is preferred to inaction without full knowledge or consideration of risks. Doctors and nurses have become attached to the pulse oximeter, believing somehow that the number displayed is less subjective and holds more predictive value than careful evaluation of the patient's respiratory status. Other pressures, such as direct to consumer marketing have made acid reflux a household term that is easily treated with over‐the‐counter medications. Considerations of the care continuum will also serve as barriers. Chest x‐rays, for example, are frequently obtained prior to admission to the hospital before the hospitalist is involved.
To overcome these limitations, the study of individual and organizational adoption of innovation might be relevant. Though it is complex and often more descriptive than proscriptive, a few salient features have emerged. Champions and opinion leaders make a difference, local culture is dominant, social networking is important, simple innovations that can be trialed on a small scale are adaptable by the user and have observable benefits, are more likely to be adopted.[56] Fortunately, the top 5 list meets many of these criteria, but also faces the daunting challenges of inertia, lack of financial incentive, inability to break with old habits, and fear of lawsuits and perceived patient/parent dissatisfaction. Ongoing evaluation, feedback, and audit will be necessary to detect and sustain change.
CONCLUSION
We have identified 5 tests or therapies overused in inpatient general pediatrics. One goal of the Choosing Wisely campaign is to begin to change social norms related to physician behavior. We hope by asking clinicians to consider doing less for common conditions in inpatient pediatrics, that they will increasingly consider the known and unanticipated risks of any medical interventions they choose to use. Finally, we would like to encourage all pediatricians to embrace the idea of good stewardship and join us in prioritizing and addressing waste and overuse as important patient safety issues as well as threats to the sustainability of our healthcare system.
Acknowledgments
The authors thank Drs. Doug Carlson, James O'Callaghan, and Karen Smith from the Society of Hospital Medicine's Pediatric and Quality and Safety Committees for their support of this effort.
Disclosure: Nothing to report.
- Eliminating waste in US health care. JAMA. 2012;307:1513–1516. , .
- Safely doing less: a missing component of the patient safety dialogue. Pediatrics. 2011;128:e1596–e1597. , , .
- To Err Is Human: Building a Safer Health System. Washington, DC: National Academy Press; 2000. , , .
- Temporal trends in rates of patient harm resulting from medical care. N Engl J Med. 2010;363:2124–2134. , , , , , .
- Screening for prostate cancer: U.S. Preventive Services Task Force recommendation statement. Ann Intern Med. 2012;157:120–134. .
- Choosing wisely: helping physicians and patients make smart decisions about their care. JAMA. 2012;307:1801–1802. , .
- The quality of ambulatory care delivered to children in the United States. N Engl J Med. 2007;357:1515–1523. , , , et al.
- National Asthma Education and Prevention Program. Expert panel report 3 (EPR‐3): guidelines for the diagnosis and management of asthma—summary report 2007. J Allergy Clin Immunol. 2007;120:S94–S138.
- The chest x‐ray and childhood acute asthma. Aust Clin Rev. 1993;13:153–156. , .
- Clinical factors associated with focal infiltrates in wheezing infants and toddlers. Clin Pediatr (Phila). 2000;39:387–393. , , , .
- Chest radiographs in the pediatric emergency department for children < or = 18 months of age with wheezing. Clin Pediatr (Phila). 1999;38:395–399. , , , .
- Clinical predictors of pneumonia among children with wheezing. Pediatrics. 2009;124:e29–e36. , , , , , .
- Reduce the rads: a quality assurance project on reducing unnecessary chest X‐rays in children with asthma. J Paediatr Child Health. 2005;41:107–111. , .
- American Academy of Pediatrics Subcommittee on Diagnosis and Management of Bronchiolitis. Diagnosis and management of bronchiolitis. Pediatrics. 2006;118:1774–1793.
- Evaluation of the utility of radiography in acute bronchiolitis. J Pediatr. 2007;150:429–433. , , , et al.
- Incidence and predisposing factors for severe disease in previously healthy term infants experiencing their first episode of bronchiolitis. Acta Paediatr. 2011;100:e17–e23. , , , et al.
- A cost effectiveness analysis of omitting radiography in diagnosis of acute bronchiolitis. Pediatr Pulmonol. 2009;44:122–127. , , , et al.
- Decreasing unnecessary utilization in acute bronchiolitis care: results from the value in inpatient pediatrics network. J Hosp Med. 2013;8:25–30. , , , et al.
- Efficacy of bronchodilator therapy in bronchiolitis. A meta‐analysis. Arch Pediatr Adolesc Med. 1996;150:1166–1172. , , , .
- Efficacy of beta2‐agonists in bronchiolitis: a reappraisal and meta‐analysis. Pediatrics. 1997;100:233–239. , .
- Bronchodilators for bronchiolitis. Cochrane Database Syst Rev. 2010;(12):CD001266. , .
- Epinephrine for bronchiolitis. Cochrane Database Syst Rev. 2011;(6):CD003123. , , , et al.
- Epinephrine and dexamethasone in children with bronchiolitis. N Engl J Med. 2009;360:2079–2089. , , , et al.
- A multicenter, randomized, double‐blind, controlled trial of nebulized epinephrine in infants with acute bronchiolitis. N Engl J Med. 2003;349:27–35. , , , et al.
- A randomized, controlled trial of the effectiveness of nebulized therapy with epinephrine compared with albuterol and saline in infants hospitalized for acute viral bronchiolitis. J Pediatr. 2002;141:818–824. , , , .
- Oral prednisolone for preschool children with acute virus‐induced wheezing. N Engl J Med. 2009;360:329–338. , , , et al.
- Glucocorticoids for acute viral bronchiolitis in infants and young children. Cochrane Database Syst Rev. 2010;(10):CD004878. , , , et al.
- Systemic corticosteroids in infant bronchiolitis: a meta‐analysis. Pediatrics. 2000;105:E44. , , , , .
- Controlled trial of oral prednisone in the emergency department treatment of children with acute asthma. Pediatrics. 1993;92:513–518. , , , .
- Methylprednisolone therapy for acute asthma in infants and toddlers: a controlled clinical trial. Pediatrics. 1990;86:350–356. , , .
- Effect of a single oral dose of prednisolone in acute childhood asthma. Lancet. 1987;1:879–882. , , , , .
- The clinical management of preterm infants with bronchiolitis. Hosp Pediatr. 2013;3:244–250. , , , , .
- Glucocorticoids and growth in asthmatic children. Pediatr Allergy Immunol. 1995;6:145–154. , .
- Adjunct corticosteroids in children hospitalized with community‐acquired pneumonia. Pediatrics. 2011;127:e255–e263. , , , , , .
- Pediatric gastroesophageal reflux disease and acid‐related conditions: trends in incidence of diagnosis and acid suppression therapy. J Med Econ. 2009;12:348–355. , , , , , .
- Healthcare costs of GERD and acid‐related conditions in pediatric patients, with comparison between histamine‐2 receptor antagonists and proton pump inhibitors. Curr Med Res Opin. 2009;25:2703–2709. , , , , , .
- Are we overprescribing antireflux medications for infants with regurgitation? Pediatrics. 2007;120:946–949. , , , .
- Proton pump inhibitor utilization patterns in infants. J Pediatr Gastroenterol Nutr. 2007;45:421–427. , , , , .
- Efficacy of proton‐pump inhibitors in children with gastroesophageal reflux disease: a systematic review. Pediatrics. 2011;127:925–935. , , , , , .
- Effectiveness and safety of proton pump inhibitors in infantile gastroesophageal reflux disease. Ann Pharmacother. 2010;44:572–576. .
- Are there risks associated with empric acid suppression treatment of infants and children suspected of having gastroesophageal reflux disease? Hosp Pediatr. 2013;3:16–23. .
- Bronchiolitis management preferences and the influence of pulse oximetry and respiratory rate on the decision to admit. Pediatrics. 2003;111:e45–e51. , , , .
- Impact of pulse oximetry and oxygen therapy on length of stay in bronchiolitis hospitalizations. Arch Pediatr Adolesc Med. 2004;158:527–530. , , , .
- Effect of oxygen supplementation on length of stay for infants hospitalized with acute viral bronchiolitis. Pediatrics. 2008;121:470–475. , .
- Oxygen therapy for bronchiolitis. Pediatrics. 2007;120:686–687; author reply 687–688. .
- Bronchiolitis‐associated hospitalizations among US children, 1980–1996. JAMA. 1999;282:1440–1446. , , , , , .
- Bronchiolitis: recent evidence on diagnosis and management. Pediatrics. 2010;125:342–349. , .
- Bronchiolitis‐associated mortality and estimates of respiratory syncytial virus‐associated deaths among US children, 1979–1997. J Infect Dis. 2001;183:16–22. , , , , .
- Observational study of two oxygen saturation targets for discharge in bronchiolitis. Arch Dis Child. 2012;97:361–363. , .
- Longitudinal assessment of hemoglobin oxygen saturation in preterm and term infants in the first six months of life. J Pediatr. 2011;159:377–383.e1. , , , et al.
- The impact of severe asthma on schoolchildren. J Asthma. 1999;36:409–417. , .
- Multi‐center, randomized trial of pulse oximetry monitoring strategies for children hospitalized for bronchiolitis. Abstract presented at: ID Week 2012; October 2012; San Diego, CA. , .
- The appropriateness method has acceptable reliability and validity for assessing overuse and underuse of surgical procedures. J Clin Epidemiol. 2012;65:1133–1143. , , , .
- Agency for Healthcare Research and Quality. HCUPnet. Kids inpatient database 2009. Available at: http://hcupnet.ahrq.gov. Accessed November 6, 2012.
- Too little? Too much? Primary care physicians' views on US health care: a brief report. Arch Intern Med. 2011;171:1582–1585. , , .
- How to implement change in clinical practice. Paediatr Respir Rev. 2003;4:340–346. .
Overuse in medicine is a significant and under‐recognized problem. Don Berwick estimated that waste accounts for at least 20% of healthcare expenditures in the United States, with overtreatment as one of the largest categories.[1] A commentary by Schroeder et al. challenged pediatricians to incorporate this knowledge into our own patient safety and quality movement.[2] Recently published data suggest that we are far from achieving the patient safety goals set forth in the Institute of Medicine's landmark To Err is Human[3] report, despite more than a decade of national, local, and regional efforts.[4] One way to reduce waste and improve patient safety is to eliminate practices of unproven benefit. Therapies or tests that may initially seem promising are often proven to be not only unhelpful but actually harmful. The recommendation of the US Preventive Services Task Force against routine screening for prostate specific antigen is an example of how a common test initially thought of as lifesaving actually increases harm.[5]
The American Board of Internal Medicine Foundation (ABIM‐F) recently announced the Choosing Wisely campaign. Through this campaign the Foundation encourages physicians, patients and other healthcare stakeholders to think and talk about medical tests and procedures that may be unnecessary.[6] The primary output of this challenge is the development of a list of 5 tests and or therapies that physicians and patients should question. The ABIM‐F approached different medical societies to develop these lists within their own specialties. The Society of Hospital Medicine (SHM) joined the Choosing Wisely campaign in April 2012, and agreed to develop a list of 5 therapies and tests for adult hospital medicine and pediatric hospital medicine. Here we present the contribution of the pediatric workgroup detailing the methodology and process for developing the list, as well as summarizing the evidence supporting each recommendation.
METHODS
In the spring of 2012, the pediatric committee of the SHM convened a workgroup of pediatric hospitalists to develop a top 5 list for the field. This workgroup was composed of experienced pediatric hospitalists representing diverse geographic locations of the United States and a mix of academic and nonacademic practice settings. The group, consisting of 4 women and 9 men, began by proposing candidate recommendations after discussion with colleagues at their different practice sites. The group was charged to maintain a focus on overuse practices that had a strong basis in evidence, were frequently encountered at their practice sites, and achieved significant consensus among their colleagues. Figure 1 shows the process map describing the method for the development of the pediatric recommendations. All workgroup participants were queried as to conflict of interest relevant to this work and none were identified.
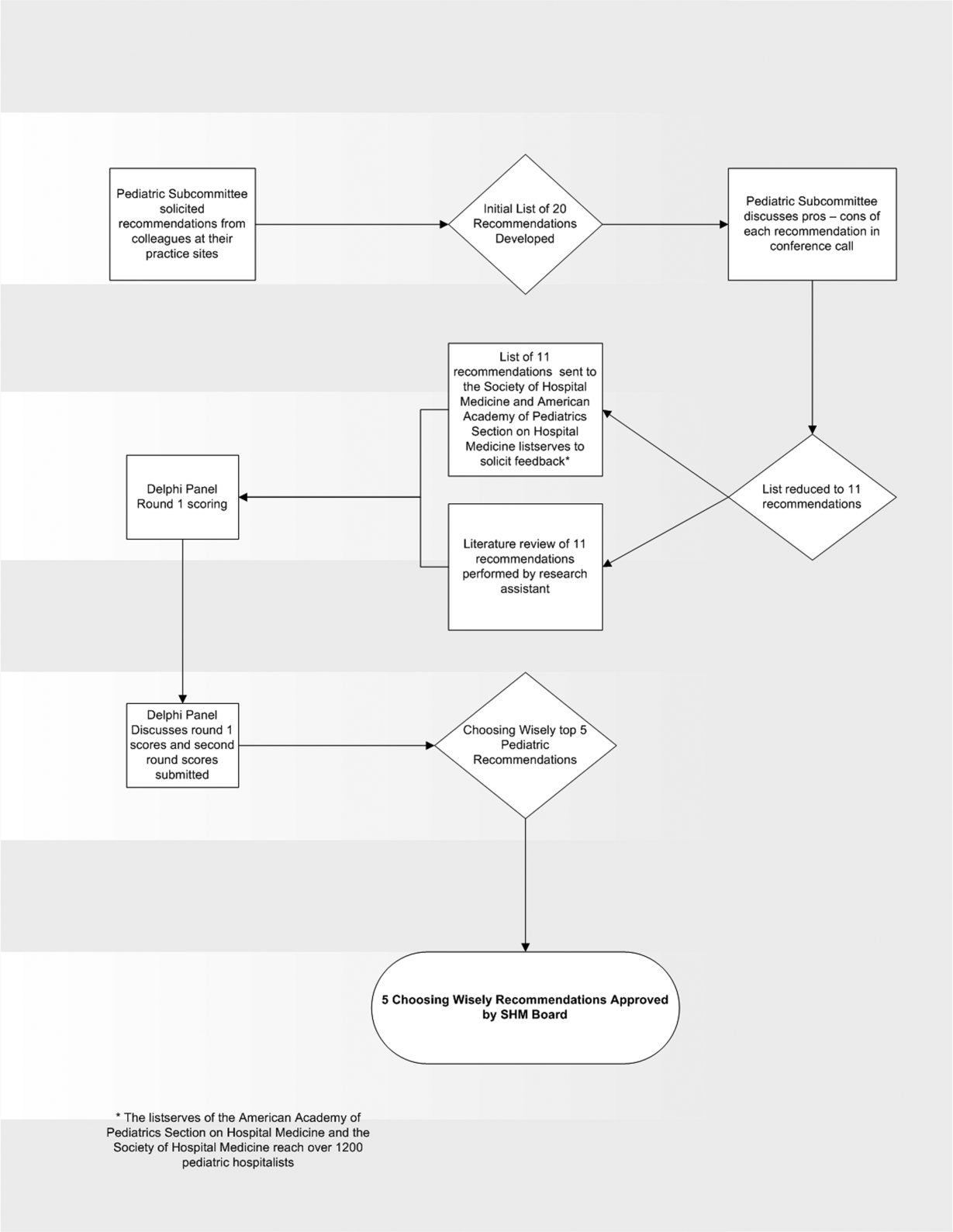
Literature Review
After the generation of the initial top 20 list, 2 reviewers conducted independent literature searches in PubMed, MEDLINE, and the Cochrane Library on the proposed topics. The reviewers also conducted generic Internet searches. Key search terms included pediatric asthma, bronchiolitis, chest radiograph, systemic corticosteroids, gastroesophageal reflux disease (GERD), infant, child, acid suppression therapy, continuous pulse oximetry, pneumonia, gastroenteritis, viral testing, blood culture, and soft tissue infections. To ensure that the reviewers included all studies relevant to the searches, they utilized broad terms. The search included all literature published through 2012, and nonEnglish language publications were included in the search. Studies selected and included in the review were based upon common criteria including whether the article discussed an evaluation of efficacy and/or utility of treatment, included a pediatric population in the guidelines or study, reviewed the harm associated with the administration of a particular test or treatment, and explored the cost associated with the test or treatment.
The Delphi Panel
Members of the workgroup formed a Delphi panel except for 1 member (R.Q.) who served as the nonvoting moderator. The members of the Delphi panel considered the results of the literature search for each recommendation along with the collated feedback from hospitalist listserves as described in Figure 1. Each panel member received a voting instrument with the candidate tests and treatments for the first round of Delphi voting. The panel utilized a modified Delphi method or the RAND Corporation (RAND)/University of California at Los Angeles (UCLA) appropriateness method as described in previous publications of quality indicator development in pediatrics.[7] Each panelist scored the candidate tests and treatments and forwarded the scores to the moderator. Subsequently, all the members of the Delphi panel met through a conference call to carry out the second round of voting. The deidentified collated results of the first round of Delphi voting were made available and discussed during the call. The moderator collated the final results, and the final 5 recommendations were those that had the highest score after the second round of Delphi voting.
Volume and Costs
During deliberations, the committee took into account the prevalence and cost rankings of our most common pediatric inpatient diagnoses. This was done using the Agency for Healthcare Research and Quality's (AHRQ) Healthcare Utilization Project (HCUP), specifically, the Kids' Inpatient Database (KID). HCUP includes the largest collection of longitudinal hospital care data in the United States, encompassing all‐payer discharge‐level information. We excluded normal newborn hospitalizations, and looked at the top 10 acute inpatient diagnoses in terms of both volume and aggregate costs.
RESULTS
The initial list of 20 candidate tests and treatments as well as the refined list of 11 recommendations can be found as electronic supplements to this publication (see Supporting Table 1 and Supporting Table 2 in the online version of this article). The format and language of the list of 11 recommendations were chosen to mesh with that typically used in the ABIM‐F Choosing Wisely campaign. During the Delphi panel, there was strong group consensus about combining items 1 and 2 (chest radiographs in asthma and bronchiolitis) into a single recommendation.
Do not order chest radiographs in children with asthma or bronchiolitis. |
Do not use bronchodilators in children with bronchiolitis. |
Do not use systemic corticosteroids in children under 2 years of age with a lower respiratory tract infection. |
Do not treat gastroesophageal reflux in infants routinely with acid suppression therapy. |
Do not use continuous pulse oximetry routinely in children with acute respiratory illness unless they are on supplemental oxygen. |
The top 5 recommendations based on the result of the second round of Delphi scoring are shown in Table 1 and described below along with a detailed evidence summary.
Do not order chest radiographs in children with asthma or bronchiolitis.
The National Heart and Lung Institute's guidelines for the management of asthma, published in 1987, recommend against routinely obtaining chest radiographs in patients with asthma or asthma exacerbations.[8] Supporting this recommendation are several studies that show a low overall yield when obtaining chest radiographs for wheezing patients.[9, 10, 11] Most relevant, studies that evaluated the clinical utility of radiographs in patients with asthma have demonstrated that they influence clinical management in less than 2% of cases.[12] A quality improvement project aimed at decreasing the rate of chest radiographs obtained in patients with asthma demonstrated that close to 60% of patients admitted to the hospital had chest radiographs performed, and that significant overall reductions can be achieved (45.3%28.9%, P=0.0005) without impacting clinical outcomes negatively.[13]
Similarly, the Subcommittee on Diagnosis and Management of Bronchiolitis of the American Academy of Pediatrics recommends against routinely obtaining radiographs during the evaluation for bronchiolitis.[14] Studies assessing the utility of chest x‐rays in these children demonstrate an even lower incidence of abnormalities (0.75%) and indicate that, despite this low incidence, physicians are more likely to treat with antibiotics when radiographs are obtained.[15] There is also evidence that chest radiographs in patients with bronchiolitis are not useful in predicting severity of illness.[16] Furthermore, cost‐effective analyses have demonstrated that omitting chest radiographs in bronchiolitis is actually cost‐effective, without compromising diagnostic accuracy.[17] In a recently published national benchmarking inpatient collaborative, Ralston et al. demonstrated that the majority of patients admitted to the hospital with bronchiolitis have chest radiographs performed at a rate of 64% (interquartile range [IQR], 54%81%).[18]
In both bronchiolitis and asthma, the elimination of unnecessary radiographs has the potential to decrease costs, reduce radiation exposure, and minimize the overuse of antibiotics that often occurs secondary to false positive results.
Do not use bronchodilators in children with bronchiolitis.
Ralston showed that 70% (IQR, 59%83%) of admitted bronchiolitis patients received bronchodilators with an average of 7.9 doses per patient (IQR, 4.69.8). National guidelines for bronchiolitis suggest a very limited role of bronchodilators in patients with bronchiolitis.[14] The first meta‐analyses of studies related to the question of ‐agonist efficacy in bronchiolitis were published in the late 1990s, revealing minimal or no treatment effects.[19, 20] Since then, further research has solidified these findings, and fairly definitive statements can be made based on a recent comprehensive meta‐analysis.[21] The pooled data do not show any effect on hospitalization rates, hospital length of stay, or other inpatient outcomes in bronchiolitis. They do show a small change in clinical scores documented in the outpatient setting, though these scores have not correlated with any detectable difference in outcomes. Routine use of ‐agonists in the inpatient setting has no proven benefit, and given the large amount of consistent data, there is no compelling reason for further study of this therapy in the inpatient setting.
Epinephrine, a combined ‐ and ‐agonist, has been extensively evaluated in bronchiolitis as well. Like albuterol, epinephrine has been reported to have no effect on hospital length of stay in bronchiolitis.[22] The issue of admission rates after epinephrine is complicated by 1 very large study that combined epinephrine with dexamethasone and reported a decreased admission rate, though only at 7 days after therapy; however, this effect was nullified after adjustment for multiple comparisons.[23] When the end point is improvement of respiratory scores, epinephrine may perform better than albuterol in studies where they are directly compared; however, there is no evidence that repeated usage of epinephrine has any impact on any clinical outcome for inpatients.[24, 25]
Do not use systemic corticosteroids in children under 2 years of age with a lower respiratory tract infection
In their summary of evidence, the Subcommittee on Diagnosis and Management of Bronchiolitis of the American Academy of Pediatrics recommends against routinely using systemic corticosteroids for infants with bronchiolitis.[14] The previously reference bronchiolitis benchmarking study demonstrated that admitted patients received steroids at a rate of 21% (IQR, 14%26%). The poor efficacy of corticosteroids in children with bronchiolitis under 2 years of age is well demonstrated in the literature. A large, blinded, randomized, controlled study compared systemic oral corticosteroids to placebo in hospitalized children 10 months to 6 years of age with viral wheezing.[26] This study showed no benefit of corticosteroids over placebo in length of stay or parental report of symptoms 1 week later. In the study, a subanalysis of children with eczema and family history of asthma also demonstrated no benefit of systemic corticosteroids. Large systematic reviews further argue that there is no effect of corticosteroids on the likelihood of admission or length of stay in infants with bronchiolitis.[27, 28] One 4‐armed prospective study of children 6 weeks to 12 months of age found no efficacy of dexamethasone over placebo.[23] There was modest benefit of dexamethasone in conjunction with racemic epinephrine; however, this benefit disappeared after adjustment for multiple comparisons. Three smaller studies showing benefit of systemic corticosteroids, however, were highly problematic. They have included older children, were retrospective, or demonstrated inconsistent results.[29, 30] A smaller study showed benefit for children over 2 years of age, but none for children under 2 years of age.[31] Premature infants are at increased risk of asthma, which typically responds well to corticosteroids as these children get older. However, a retrospective study of premature infants under 2 years of age with bronchiolitis demonstrated no association between corticosteroid use and length of stay, even in the subset of premature infants responding to albuterol.[32]
Systemic corticosteroid use in children is not harmless. Children under 2 years of age are especially vulnerable to the decreased growth velocity seen as a side effect of systemic corticosteroids.[33] Corticosteroids may also negatively impact the course of infectious illness. For instance, in children hospitalized with pneumonia but not receiving ‐agonists (ie, patients who are unlikely to have asthma), length of stay is prolonged and readmission is higher in those who receive corticosteroids.[34]
Do not treat gastroesophageal reflux in infants routinely with acid suppression therapy.
From 2000 to 2005, the incidence of infants diagnosed with gastroeshopaheal reflux (GER) tripled (3.4%12.3%), and the use of proton pump inhibitors (PPIs) doubled (31.5%62.6%).[35] Patients diagnosed with GER and treated with antireflux medication incurred 1.8 times higher healthcare costs in 1 study compared to healthy controls.[36] Though common, the use of acid suppressive medications in infants lacks evidence for efficacy in the majority of the clinical scenarios in which they are prescribed.[37, 38] PPIs have failed to outperform placebo for typical infant reflux, which is generally developmental and not pathologic.[39, 40] Furthermore, prompted by findings in adults, multiple pediatric investigators have now catalogued the potential risks associated with acid blockade in children in multiple clinical settings. Specifically, increased risk of pneumonia has been documented in inpatients and outpatients, and increased risk of necrotizing enterocolitis and other serious infections have been documented in intensive care unit settings.[41] In the absence of data supporting efficacy and given the emerging data on risk, empiric acid suppression in infants with reflux is wasteful and potentially harmful.
Do not use continuous pulse oximetry routinely in children with acute respiratory illness unless they are on supplemental oxygen.
Pulse oximetry use has become widespread in the management of infants with bronchiolitis and likely accounts for the dramatic increase in bronchiolitis hospitalization rates in recent years.[14, 42, 43, 44, 45, 46, 47] Despite this increase in hospitalization rate, there was no change in mortality from bronchiolitis between 1979 and 1997.[48] The continuous monitoring of oxygen saturations in hospitalized infants with bronchiolitis may lead to overdiagnosis of hypoxemia and subsequent oxygen use that is of no apparent benefit to the child. Schroeder et al. demonstrated that 26% of a sample of infants hospitalized with bronchiolitis had a prolonged length of stay because of a perceived need for oxygen based on pulse oximetry readings.[43] Unger and Cunningham showed that the need for oxygen was the final determinant of length of stay in 58% of cases, and Cunningham and Murray suggested that using an oxygen saturation cutoff of 94% instead of 90% might increase the length of stay by 22 hours.[44, 49]
It has been previously shown that hypoxia is normative in infants. Healthy infants experience multiple episodes of SpO2 90% while sleeping.[50] This finding strengthens the notion that detection of low saturations in infants convalescing from bronchiolitis may simply reflect overdiagnosis. Among children with chronic severe asthma, who presumably have experienced episodes of hypoxia throughout childhood, there is no difference in school performance compared to healthy controls.[51]
The practice parameter on bronchiolitis from the American Academy of Pediatrics states: as the child's clinical course improves, continuous measurement of SpO2 is not routinely needed, which is a recommendation based on expert consensus.[14] There is at least one ongoing randomized trial comparing the use of continuous versus intermittent pulse oximetry in hospitalized infants with bronchiolitis who are weaned off oxygen (
DISCUSSION
Berwick and Hackbarth define overtreatment as: waste that comes from subjecting patients to care that, according to sound science and the patients' own preferences, cannot possibly help themcare rooted in outmoded habits, supply‐driven behaviors, and ignoring science.[1] With this project, we tried to capture common clinical sources of waste in the inpatient pediatric setting. This is an inherently difficult project because of the absence of solid evidence to inform every decision point in medicine. Although there is always room for improvement in our evidence base, our group intentionally gravitated to areas where the evidence was robust.
The primary strength of this work is the use of the RAND/UCLA appropriateness method or modified Delphi method. Several publications have validated this methodology as a sound strategy to assess quality indicators and issues related to overuse.[7, 53] To our knowledge, we are the first group to report the use of this methodology to develop a list such as the list reported here.
There were some challenges inherent to this project that can be considered limitations of the work. One perceived limitation of our list is the heavy concentration on respiratory diagnoses, especially bronchiolitis and asthma. We do not feel this is a genuine limitation, as the recommendations were partly driven by volume and costs as assessed by the KID database. Among the top 10 acute inpatient diagnoses in pediatrics, respiratory diagnoses are the most common, including bronchiolitis, pneumonia, and asthma. Pneumonia or bronchiolitis has been the most common medical diagnosis in inpatient pediatrics for the past decade, and both are always in the top 10 for costs as well.[54] Thus, the impact of decreasing overuse for these conditions will be highly significant from a simple volume standpoint.
The primary limitation of this work is the lack of implementation strategies. Although the Choosing Wisely campaign has plans for dissemination of the lists, compliance with the recommendations may be suboptimal. Although the development process followed an accepted methodology, shortcomings include the lack of wide, local, multidisciplinary (including parents or caretakers) consultation. Other barriers to compliance with these recommendations exist. Despite evidence that bronchiolitis is a benign self‐limited disease that does not respond to bronchodilators and steroids, the drive to identify and correct all abnormalities, such as wheezing or low oxygen saturation in a nontoxic infant with bronchiolitis, seems to trump the obligation to do no harm in daily practice.[55] This behavior may result from pressure by patients, families, nurses, or peers and is deeply embedded in our medical culture, where action is preferred to inaction without full knowledge or consideration of risks. Doctors and nurses have become attached to the pulse oximeter, believing somehow that the number displayed is less subjective and holds more predictive value than careful evaluation of the patient's respiratory status. Other pressures, such as direct to consumer marketing have made acid reflux a household term that is easily treated with over‐the‐counter medications. Considerations of the care continuum will also serve as barriers. Chest x‐rays, for example, are frequently obtained prior to admission to the hospital before the hospitalist is involved.
To overcome these limitations, the study of individual and organizational adoption of innovation might be relevant. Though it is complex and often more descriptive than proscriptive, a few salient features have emerged. Champions and opinion leaders make a difference, local culture is dominant, social networking is important, simple innovations that can be trialed on a small scale are adaptable by the user and have observable benefits, are more likely to be adopted.[56] Fortunately, the top 5 list meets many of these criteria, but also faces the daunting challenges of inertia, lack of financial incentive, inability to break with old habits, and fear of lawsuits and perceived patient/parent dissatisfaction. Ongoing evaluation, feedback, and audit will be necessary to detect and sustain change.
CONCLUSION
We have identified 5 tests or therapies overused in inpatient general pediatrics. One goal of the Choosing Wisely campaign is to begin to change social norms related to physician behavior. We hope by asking clinicians to consider doing less for common conditions in inpatient pediatrics, that they will increasingly consider the known and unanticipated risks of any medical interventions they choose to use. Finally, we would like to encourage all pediatricians to embrace the idea of good stewardship and join us in prioritizing and addressing waste and overuse as important patient safety issues as well as threats to the sustainability of our healthcare system.
Acknowledgments
The authors thank Drs. Doug Carlson, James O'Callaghan, and Karen Smith from the Society of Hospital Medicine's Pediatric and Quality and Safety Committees for their support of this effort.
Disclosure: Nothing to report.
Overuse in medicine is a significant and under‐recognized problem. Don Berwick estimated that waste accounts for at least 20% of healthcare expenditures in the United States, with overtreatment as one of the largest categories.[1] A commentary by Schroeder et al. challenged pediatricians to incorporate this knowledge into our own patient safety and quality movement.[2] Recently published data suggest that we are far from achieving the patient safety goals set forth in the Institute of Medicine's landmark To Err is Human[3] report, despite more than a decade of national, local, and regional efforts.[4] One way to reduce waste and improve patient safety is to eliminate practices of unproven benefit. Therapies or tests that may initially seem promising are often proven to be not only unhelpful but actually harmful. The recommendation of the US Preventive Services Task Force against routine screening for prostate specific antigen is an example of how a common test initially thought of as lifesaving actually increases harm.[5]
The American Board of Internal Medicine Foundation (ABIM‐F) recently announced the Choosing Wisely campaign. Through this campaign the Foundation encourages physicians, patients and other healthcare stakeholders to think and talk about medical tests and procedures that may be unnecessary.[6] The primary output of this challenge is the development of a list of 5 tests and or therapies that physicians and patients should question. The ABIM‐F approached different medical societies to develop these lists within their own specialties. The Society of Hospital Medicine (SHM) joined the Choosing Wisely campaign in April 2012, and agreed to develop a list of 5 therapies and tests for adult hospital medicine and pediatric hospital medicine. Here we present the contribution of the pediatric workgroup detailing the methodology and process for developing the list, as well as summarizing the evidence supporting each recommendation.
METHODS
In the spring of 2012, the pediatric committee of the SHM convened a workgroup of pediatric hospitalists to develop a top 5 list for the field. This workgroup was composed of experienced pediatric hospitalists representing diverse geographic locations of the United States and a mix of academic and nonacademic practice settings. The group, consisting of 4 women and 9 men, began by proposing candidate recommendations after discussion with colleagues at their different practice sites. The group was charged to maintain a focus on overuse practices that had a strong basis in evidence, were frequently encountered at their practice sites, and achieved significant consensus among their colleagues. Figure 1 shows the process map describing the method for the development of the pediatric recommendations. All workgroup participants were queried as to conflict of interest relevant to this work and none were identified.
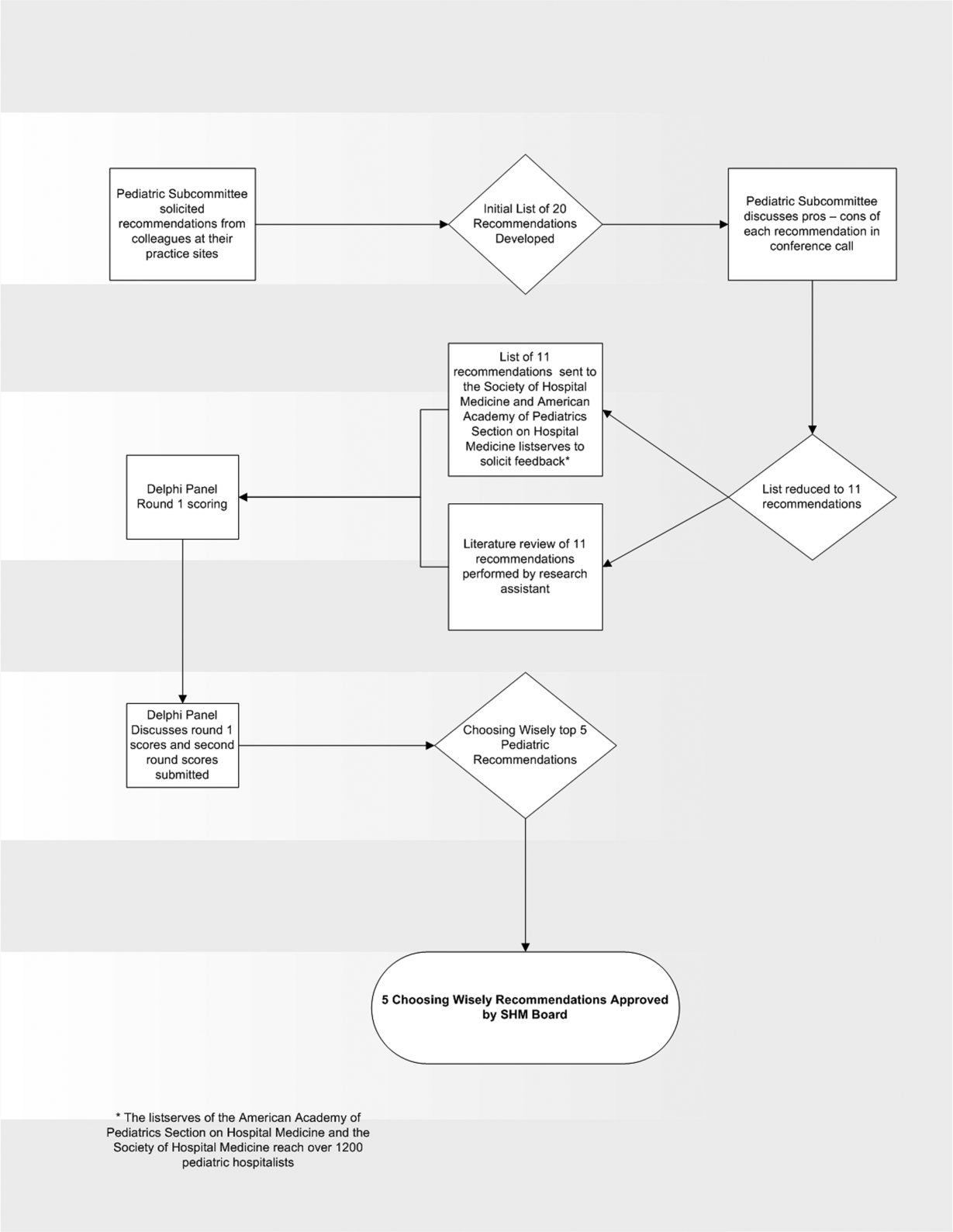
Literature Review
After the generation of the initial top 20 list, 2 reviewers conducted independent literature searches in PubMed, MEDLINE, and the Cochrane Library on the proposed topics. The reviewers also conducted generic Internet searches. Key search terms included pediatric asthma, bronchiolitis, chest radiograph, systemic corticosteroids, gastroesophageal reflux disease (GERD), infant, child, acid suppression therapy, continuous pulse oximetry, pneumonia, gastroenteritis, viral testing, blood culture, and soft tissue infections. To ensure that the reviewers included all studies relevant to the searches, they utilized broad terms. The search included all literature published through 2012, and nonEnglish language publications were included in the search. Studies selected and included in the review were based upon common criteria including whether the article discussed an evaluation of efficacy and/or utility of treatment, included a pediatric population in the guidelines or study, reviewed the harm associated with the administration of a particular test or treatment, and explored the cost associated with the test or treatment.
The Delphi Panel
Members of the workgroup formed a Delphi panel except for 1 member (R.Q.) who served as the nonvoting moderator. The members of the Delphi panel considered the results of the literature search for each recommendation along with the collated feedback from hospitalist listserves as described in Figure 1. Each panel member received a voting instrument with the candidate tests and treatments for the first round of Delphi voting. The panel utilized a modified Delphi method or the RAND Corporation (RAND)/University of California at Los Angeles (UCLA) appropriateness method as described in previous publications of quality indicator development in pediatrics.[7] Each panelist scored the candidate tests and treatments and forwarded the scores to the moderator. Subsequently, all the members of the Delphi panel met through a conference call to carry out the second round of voting. The deidentified collated results of the first round of Delphi voting were made available and discussed during the call. The moderator collated the final results, and the final 5 recommendations were those that had the highest score after the second round of Delphi voting.
Volume and Costs
During deliberations, the committee took into account the prevalence and cost rankings of our most common pediatric inpatient diagnoses. This was done using the Agency for Healthcare Research and Quality's (AHRQ) Healthcare Utilization Project (HCUP), specifically, the Kids' Inpatient Database (KID). HCUP includes the largest collection of longitudinal hospital care data in the United States, encompassing all‐payer discharge‐level information. We excluded normal newborn hospitalizations, and looked at the top 10 acute inpatient diagnoses in terms of both volume and aggregate costs.
RESULTS
The initial list of 20 candidate tests and treatments as well as the refined list of 11 recommendations can be found as electronic supplements to this publication (see Supporting Table 1 and Supporting Table 2 in the online version of this article). The format and language of the list of 11 recommendations were chosen to mesh with that typically used in the ABIM‐F Choosing Wisely campaign. During the Delphi panel, there was strong group consensus about combining items 1 and 2 (chest radiographs in asthma and bronchiolitis) into a single recommendation.
Do not order chest radiographs in children with asthma or bronchiolitis. |
Do not use bronchodilators in children with bronchiolitis. |
Do not use systemic corticosteroids in children under 2 years of age with a lower respiratory tract infection. |
Do not treat gastroesophageal reflux in infants routinely with acid suppression therapy. |
Do not use continuous pulse oximetry routinely in children with acute respiratory illness unless they are on supplemental oxygen. |
The top 5 recommendations based on the result of the second round of Delphi scoring are shown in Table 1 and described below along with a detailed evidence summary.
Do not order chest radiographs in children with asthma or bronchiolitis.
The National Heart and Lung Institute's guidelines for the management of asthma, published in 1987, recommend against routinely obtaining chest radiographs in patients with asthma or asthma exacerbations.[8] Supporting this recommendation are several studies that show a low overall yield when obtaining chest radiographs for wheezing patients.[9, 10, 11] Most relevant, studies that evaluated the clinical utility of radiographs in patients with asthma have demonstrated that they influence clinical management in less than 2% of cases.[12] A quality improvement project aimed at decreasing the rate of chest radiographs obtained in patients with asthma demonstrated that close to 60% of patients admitted to the hospital had chest radiographs performed, and that significant overall reductions can be achieved (45.3%28.9%, P=0.0005) without impacting clinical outcomes negatively.[13]
Similarly, the Subcommittee on Diagnosis and Management of Bronchiolitis of the American Academy of Pediatrics recommends against routinely obtaining radiographs during the evaluation for bronchiolitis.[14] Studies assessing the utility of chest x‐rays in these children demonstrate an even lower incidence of abnormalities (0.75%) and indicate that, despite this low incidence, physicians are more likely to treat with antibiotics when radiographs are obtained.[15] There is also evidence that chest radiographs in patients with bronchiolitis are not useful in predicting severity of illness.[16] Furthermore, cost‐effective analyses have demonstrated that omitting chest radiographs in bronchiolitis is actually cost‐effective, without compromising diagnostic accuracy.[17] In a recently published national benchmarking inpatient collaborative, Ralston et al. demonstrated that the majority of patients admitted to the hospital with bronchiolitis have chest radiographs performed at a rate of 64% (interquartile range [IQR], 54%81%).[18]
In both bronchiolitis and asthma, the elimination of unnecessary radiographs has the potential to decrease costs, reduce radiation exposure, and minimize the overuse of antibiotics that often occurs secondary to false positive results.
Do not use bronchodilators in children with bronchiolitis.
Ralston showed that 70% (IQR, 59%83%) of admitted bronchiolitis patients received bronchodilators with an average of 7.9 doses per patient (IQR, 4.69.8). National guidelines for bronchiolitis suggest a very limited role of bronchodilators in patients with bronchiolitis.[14] The first meta‐analyses of studies related to the question of ‐agonist efficacy in bronchiolitis were published in the late 1990s, revealing minimal or no treatment effects.[19, 20] Since then, further research has solidified these findings, and fairly definitive statements can be made based on a recent comprehensive meta‐analysis.[21] The pooled data do not show any effect on hospitalization rates, hospital length of stay, or other inpatient outcomes in bronchiolitis. They do show a small change in clinical scores documented in the outpatient setting, though these scores have not correlated with any detectable difference in outcomes. Routine use of ‐agonists in the inpatient setting has no proven benefit, and given the large amount of consistent data, there is no compelling reason for further study of this therapy in the inpatient setting.
Epinephrine, a combined ‐ and ‐agonist, has been extensively evaluated in bronchiolitis as well. Like albuterol, epinephrine has been reported to have no effect on hospital length of stay in bronchiolitis.[22] The issue of admission rates after epinephrine is complicated by 1 very large study that combined epinephrine with dexamethasone and reported a decreased admission rate, though only at 7 days after therapy; however, this effect was nullified after adjustment for multiple comparisons.[23] When the end point is improvement of respiratory scores, epinephrine may perform better than albuterol in studies where they are directly compared; however, there is no evidence that repeated usage of epinephrine has any impact on any clinical outcome for inpatients.[24, 25]
Do not use systemic corticosteroids in children under 2 years of age with a lower respiratory tract infection
In their summary of evidence, the Subcommittee on Diagnosis and Management of Bronchiolitis of the American Academy of Pediatrics recommends against routinely using systemic corticosteroids for infants with bronchiolitis.[14] The previously reference bronchiolitis benchmarking study demonstrated that admitted patients received steroids at a rate of 21% (IQR, 14%26%). The poor efficacy of corticosteroids in children with bronchiolitis under 2 years of age is well demonstrated in the literature. A large, blinded, randomized, controlled study compared systemic oral corticosteroids to placebo in hospitalized children 10 months to 6 years of age with viral wheezing.[26] This study showed no benefit of corticosteroids over placebo in length of stay or parental report of symptoms 1 week later. In the study, a subanalysis of children with eczema and family history of asthma also demonstrated no benefit of systemic corticosteroids. Large systematic reviews further argue that there is no effect of corticosteroids on the likelihood of admission or length of stay in infants with bronchiolitis.[27, 28] One 4‐armed prospective study of children 6 weeks to 12 months of age found no efficacy of dexamethasone over placebo.[23] There was modest benefit of dexamethasone in conjunction with racemic epinephrine; however, this benefit disappeared after adjustment for multiple comparisons. Three smaller studies showing benefit of systemic corticosteroids, however, were highly problematic. They have included older children, were retrospective, or demonstrated inconsistent results.[29, 30] A smaller study showed benefit for children over 2 years of age, but none for children under 2 years of age.[31] Premature infants are at increased risk of asthma, which typically responds well to corticosteroids as these children get older. However, a retrospective study of premature infants under 2 years of age with bronchiolitis demonstrated no association between corticosteroid use and length of stay, even in the subset of premature infants responding to albuterol.[32]
Systemic corticosteroid use in children is not harmless. Children under 2 years of age are especially vulnerable to the decreased growth velocity seen as a side effect of systemic corticosteroids.[33] Corticosteroids may also negatively impact the course of infectious illness. For instance, in children hospitalized with pneumonia but not receiving ‐agonists (ie, patients who are unlikely to have asthma), length of stay is prolonged and readmission is higher in those who receive corticosteroids.[34]
Do not treat gastroesophageal reflux in infants routinely with acid suppression therapy.
From 2000 to 2005, the incidence of infants diagnosed with gastroeshopaheal reflux (GER) tripled (3.4%12.3%), and the use of proton pump inhibitors (PPIs) doubled (31.5%62.6%).[35] Patients diagnosed with GER and treated with antireflux medication incurred 1.8 times higher healthcare costs in 1 study compared to healthy controls.[36] Though common, the use of acid suppressive medications in infants lacks evidence for efficacy in the majority of the clinical scenarios in which they are prescribed.[37, 38] PPIs have failed to outperform placebo for typical infant reflux, which is generally developmental and not pathologic.[39, 40] Furthermore, prompted by findings in adults, multiple pediatric investigators have now catalogued the potential risks associated with acid blockade in children in multiple clinical settings. Specifically, increased risk of pneumonia has been documented in inpatients and outpatients, and increased risk of necrotizing enterocolitis and other serious infections have been documented in intensive care unit settings.[41] In the absence of data supporting efficacy and given the emerging data on risk, empiric acid suppression in infants with reflux is wasteful and potentially harmful.
Do not use continuous pulse oximetry routinely in children with acute respiratory illness unless they are on supplemental oxygen.
Pulse oximetry use has become widespread in the management of infants with bronchiolitis and likely accounts for the dramatic increase in bronchiolitis hospitalization rates in recent years.[14, 42, 43, 44, 45, 46, 47] Despite this increase in hospitalization rate, there was no change in mortality from bronchiolitis between 1979 and 1997.[48] The continuous monitoring of oxygen saturations in hospitalized infants with bronchiolitis may lead to overdiagnosis of hypoxemia and subsequent oxygen use that is of no apparent benefit to the child. Schroeder et al. demonstrated that 26% of a sample of infants hospitalized with bronchiolitis had a prolonged length of stay because of a perceived need for oxygen based on pulse oximetry readings.[43] Unger and Cunningham showed that the need for oxygen was the final determinant of length of stay in 58% of cases, and Cunningham and Murray suggested that using an oxygen saturation cutoff of 94% instead of 90% might increase the length of stay by 22 hours.[44, 49]
It has been previously shown that hypoxia is normative in infants. Healthy infants experience multiple episodes of SpO2 90% while sleeping.[50] This finding strengthens the notion that detection of low saturations in infants convalescing from bronchiolitis may simply reflect overdiagnosis. Among children with chronic severe asthma, who presumably have experienced episodes of hypoxia throughout childhood, there is no difference in school performance compared to healthy controls.[51]
The practice parameter on bronchiolitis from the American Academy of Pediatrics states: as the child's clinical course improves, continuous measurement of SpO2 is not routinely needed, which is a recommendation based on expert consensus.[14] There is at least one ongoing randomized trial comparing the use of continuous versus intermittent pulse oximetry in hospitalized infants with bronchiolitis who are weaned off oxygen (
DISCUSSION
Berwick and Hackbarth define overtreatment as: waste that comes from subjecting patients to care that, according to sound science and the patients' own preferences, cannot possibly help themcare rooted in outmoded habits, supply‐driven behaviors, and ignoring science.[1] With this project, we tried to capture common clinical sources of waste in the inpatient pediatric setting. This is an inherently difficult project because of the absence of solid evidence to inform every decision point in medicine. Although there is always room for improvement in our evidence base, our group intentionally gravitated to areas where the evidence was robust.
The primary strength of this work is the use of the RAND/UCLA appropriateness method or modified Delphi method. Several publications have validated this methodology as a sound strategy to assess quality indicators and issues related to overuse.[7, 53] To our knowledge, we are the first group to report the use of this methodology to develop a list such as the list reported here.
There were some challenges inherent to this project that can be considered limitations of the work. One perceived limitation of our list is the heavy concentration on respiratory diagnoses, especially bronchiolitis and asthma. We do not feel this is a genuine limitation, as the recommendations were partly driven by volume and costs as assessed by the KID database. Among the top 10 acute inpatient diagnoses in pediatrics, respiratory diagnoses are the most common, including bronchiolitis, pneumonia, and asthma. Pneumonia or bronchiolitis has been the most common medical diagnosis in inpatient pediatrics for the past decade, and both are always in the top 10 for costs as well.[54] Thus, the impact of decreasing overuse for these conditions will be highly significant from a simple volume standpoint.
The primary limitation of this work is the lack of implementation strategies. Although the Choosing Wisely campaign has plans for dissemination of the lists, compliance with the recommendations may be suboptimal. Although the development process followed an accepted methodology, shortcomings include the lack of wide, local, multidisciplinary (including parents or caretakers) consultation. Other barriers to compliance with these recommendations exist. Despite evidence that bronchiolitis is a benign self‐limited disease that does not respond to bronchodilators and steroids, the drive to identify and correct all abnormalities, such as wheezing or low oxygen saturation in a nontoxic infant with bronchiolitis, seems to trump the obligation to do no harm in daily practice.[55] This behavior may result from pressure by patients, families, nurses, or peers and is deeply embedded in our medical culture, where action is preferred to inaction without full knowledge or consideration of risks. Doctors and nurses have become attached to the pulse oximeter, believing somehow that the number displayed is less subjective and holds more predictive value than careful evaluation of the patient's respiratory status. Other pressures, such as direct to consumer marketing have made acid reflux a household term that is easily treated with over‐the‐counter medications. Considerations of the care continuum will also serve as barriers. Chest x‐rays, for example, are frequently obtained prior to admission to the hospital before the hospitalist is involved.
To overcome these limitations, the study of individual and organizational adoption of innovation might be relevant. Though it is complex and often more descriptive than proscriptive, a few salient features have emerged. Champions and opinion leaders make a difference, local culture is dominant, social networking is important, simple innovations that can be trialed on a small scale are adaptable by the user and have observable benefits, are more likely to be adopted.[56] Fortunately, the top 5 list meets many of these criteria, but also faces the daunting challenges of inertia, lack of financial incentive, inability to break with old habits, and fear of lawsuits and perceived patient/parent dissatisfaction. Ongoing evaluation, feedback, and audit will be necessary to detect and sustain change.
CONCLUSION
We have identified 5 tests or therapies overused in inpatient general pediatrics. One goal of the Choosing Wisely campaign is to begin to change social norms related to physician behavior. We hope by asking clinicians to consider doing less for common conditions in inpatient pediatrics, that they will increasingly consider the known and unanticipated risks of any medical interventions they choose to use. Finally, we would like to encourage all pediatricians to embrace the idea of good stewardship and join us in prioritizing and addressing waste and overuse as important patient safety issues as well as threats to the sustainability of our healthcare system.
Acknowledgments
The authors thank Drs. Doug Carlson, James O'Callaghan, and Karen Smith from the Society of Hospital Medicine's Pediatric and Quality and Safety Committees for their support of this effort.
Disclosure: Nothing to report.
- Eliminating waste in US health care. JAMA. 2012;307:1513–1516. , .
- Safely doing less: a missing component of the patient safety dialogue. Pediatrics. 2011;128:e1596–e1597. , , .
- To Err Is Human: Building a Safer Health System. Washington, DC: National Academy Press; 2000. , , .
- Temporal trends in rates of patient harm resulting from medical care. N Engl J Med. 2010;363:2124–2134. , , , , , .
- Screening for prostate cancer: U.S. Preventive Services Task Force recommendation statement. Ann Intern Med. 2012;157:120–134. .
- Choosing wisely: helping physicians and patients make smart decisions about their care. JAMA. 2012;307:1801–1802. , .
- The quality of ambulatory care delivered to children in the United States. N Engl J Med. 2007;357:1515–1523. , , , et al.
- National Asthma Education and Prevention Program. Expert panel report 3 (EPR‐3): guidelines for the diagnosis and management of asthma—summary report 2007. J Allergy Clin Immunol. 2007;120:S94–S138.
- The chest x‐ray and childhood acute asthma. Aust Clin Rev. 1993;13:153–156. , .
- Clinical factors associated with focal infiltrates in wheezing infants and toddlers. Clin Pediatr (Phila). 2000;39:387–393. , , , .
- Chest radiographs in the pediatric emergency department for children < or = 18 months of age with wheezing. Clin Pediatr (Phila). 1999;38:395–399. , , , .
- Clinical predictors of pneumonia among children with wheezing. Pediatrics. 2009;124:e29–e36. , , , , , .
- Reduce the rads: a quality assurance project on reducing unnecessary chest X‐rays in children with asthma. J Paediatr Child Health. 2005;41:107–111. , .
- American Academy of Pediatrics Subcommittee on Diagnosis and Management of Bronchiolitis. Diagnosis and management of bronchiolitis. Pediatrics. 2006;118:1774–1793.
- Evaluation of the utility of radiography in acute bronchiolitis. J Pediatr. 2007;150:429–433. , , , et al.
- Incidence and predisposing factors for severe disease in previously healthy term infants experiencing their first episode of bronchiolitis. Acta Paediatr. 2011;100:e17–e23. , , , et al.
- A cost effectiveness analysis of omitting radiography in diagnosis of acute bronchiolitis. Pediatr Pulmonol. 2009;44:122–127. , , , et al.
- Decreasing unnecessary utilization in acute bronchiolitis care: results from the value in inpatient pediatrics network. J Hosp Med. 2013;8:25–30. , , , et al.
- Efficacy of bronchodilator therapy in bronchiolitis. A meta‐analysis. Arch Pediatr Adolesc Med. 1996;150:1166–1172. , , , .
- Efficacy of beta2‐agonists in bronchiolitis: a reappraisal and meta‐analysis. Pediatrics. 1997;100:233–239. , .
- Bronchodilators for bronchiolitis. Cochrane Database Syst Rev. 2010;(12):CD001266. , .
- Epinephrine for bronchiolitis. Cochrane Database Syst Rev. 2011;(6):CD003123. , , , et al.
- Epinephrine and dexamethasone in children with bronchiolitis. N Engl J Med. 2009;360:2079–2089. , , , et al.
- A multicenter, randomized, double‐blind, controlled trial of nebulized epinephrine in infants with acute bronchiolitis. N Engl J Med. 2003;349:27–35. , , , et al.
- A randomized, controlled trial of the effectiveness of nebulized therapy with epinephrine compared with albuterol and saline in infants hospitalized for acute viral bronchiolitis. J Pediatr. 2002;141:818–824. , , , .
- Oral prednisolone for preschool children with acute virus‐induced wheezing. N Engl J Med. 2009;360:329–338. , , , et al.
- Glucocorticoids for acute viral bronchiolitis in infants and young children. Cochrane Database Syst Rev. 2010;(10):CD004878. , , , et al.
- Systemic corticosteroids in infant bronchiolitis: a meta‐analysis. Pediatrics. 2000;105:E44. , , , , .
- Controlled trial of oral prednisone in the emergency department treatment of children with acute asthma. Pediatrics. 1993;92:513–518. , , , .
- Methylprednisolone therapy for acute asthma in infants and toddlers: a controlled clinical trial. Pediatrics. 1990;86:350–356. , , .
- Effect of a single oral dose of prednisolone in acute childhood asthma. Lancet. 1987;1:879–882. , , , , .
- The clinical management of preterm infants with bronchiolitis. Hosp Pediatr. 2013;3:244–250. , , , , .
- Glucocorticoids and growth in asthmatic children. Pediatr Allergy Immunol. 1995;6:145–154. , .
- Adjunct corticosteroids in children hospitalized with community‐acquired pneumonia. Pediatrics. 2011;127:e255–e263. , , , , , .
- Pediatric gastroesophageal reflux disease and acid‐related conditions: trends in incidence of diagnosis and acid suppression therapy. J Med Econ. 2009;12:348–355. , , , , , .
- Healthcare costs of GERD and acid‐related conditions in pediatric patients, with comparison between histamine‐2 receptor antagonists and proton pump inhibitors. Curr Med Res Opin. 2009;25:2703–2709. , , , , , .
- Are we overprescribing antireflux medications for infants with regurgitation? Pediatrics. 2007;120:946–949. , , , .
- Proton pump inhibitor utilization patterns in infants. J Pediatr Gastroenterol Nutr. 2007;45:421–427. , , , , .
- Efficacy of proton‐pump inhibitors in children with gastroesophageal reflux disease: a systematic review. Pediatrics. 2011;127:925–935. , , , , , .
- Effectiveness and safety of proton pump inhibitors in infantile gastroesophageal reflux disease. Ann Pharmacother. 2010;44:572–576. .
- Are there risks associated with empric acid suppression treatment of infants and children suspected of having gastroesophageal reflux disease? Hosp Pediatr. 2013;3:16–23. .
- Bronchiolitis management preferences and the influence of pulse oximetry and respiratory rate on the decision to admit. Pediatrics. 2003;111:e45–e51. , , , .
- Impact of pulse oximetry and oxygen therapy on length of stay in bronchiolitis hospitalizations. Arch Pediatr Adolesc Med. 2004;158:527–530. , , , .
- Effect of oxygen supplementation on length of stay for infants hospitalized with acute viral bronchiolitis. Pediatrics. 2008;121:470–475. , .
- Oxygen therapy for bronchiolitis. Pediatrics. 2007;120:686–687; author reply 687–688. .
- Bronchiolitis‐associated hospitalizations among US children, 1980–1996. JAMA. 1999;282:1440–1446. , , , , , .
- Bronchiolitis: recent evidence on diagnosis and management. Pediatrics. 2010;125:342–349. , .
- Bronchiolitis‐associated mortality and estimates of respiratory syncytial virus‐associated deaths among US children, 1979–1997. J Infect Dis. 2001;183:16–22. , , , , .
- Observational study of two oxygen saturation targets for discharge in bronchiolitis. Arch Dis Child. 2012;97:361–363. , .
- Longitudinal assessment of hemoglobin oxygen saturation in preterm and term infants in the first six months of life. J Pediatr. 2011;159:377–383.e1. , , , et al.
- The impact of severe asthma on schoolchildren. J Asthma. 1999;36:409–417. , .
- Multi‐center, randomized trial of pulse oximetry monitoring strategies for children hospitalized for bronchiolitis. Abstract presented at: ID Week 2012; October 2012; San Diego, CA. , .
- The appropriateness method has acceptable reliability and validity for assessing overuse and underuse of surgical procedures. J Clin Epidemiol. 2012;65:1133–1143. , , , .
- Agency for Healthcare Research and Quality. HCUPnet. Kids inpatient database 2009. Available at: http://hcupnet.ahrq.gov. Accessed November 6, 2012.
- Too little? Too much? Primary care physicians' views on US health care: a brief report. Arch Intern Med. 2011;171:1582–1585. , , .
- How to implement change in clinical practice. Paediatr Respir Rev. 2003;4:340–346. .
- Eliminating waste in US health care. JAMA. 2012;307:1513–1516. , .
- Safely doing less: a missing component of the patient safety dialogue. Pediatrics. 2011;128:e1596–e1597. , , .
- To Err Is Human: Building a Safer Health System. Washington, DC: National Academy Press; 2000. , , .
- Temporal trends in rates of patient harm resulting from medical care. N Engl J Med. 2010;363:2124–2134. , , , , , .
- Screening for prostate cancer: U.S. Preventive Services Task Force recommendation statement. Ann Intern Med. 2012;157:120–134. .
- Choosing wisely: helping physicians and patients make smart decisions about their care. JAMA. 2012;307:1801–1802. , .
- The quality of ambulatory care delivered to children in the United States. N Engl J Med. 2007;357:1515–1523. , , , et al.
- National Asthma Education and Prevention Program. Expert panel report 3 (EPR‐3): guidelines for the diagnosis and management of asthma—summary report 2007. J Allergy Clin Immunol. 2007;120:S94–S138.
- The chest x‐ray and childhood acute asthma. Aust Clin Rev. 1993;13:153–156. , .
- Clinical factors associated with focal infiltrates in wheezing infants and toddlers. Clin Pediatr (Phila). 2000;39:387–393. , , , .
- Chest radiographs in the pediatric emergency department for children < or = 18 months of age with wheezing. Clin Pediatr (Phila). 1999;38:395–399. , , , .
- Clinical predictors of pneumonia among children with wheezing. Pediatrics. 2009;124:e29–e36. , , , , , .
- Reduce the rads: a quality assurance project on reducing unnecessary chest X‐rays in children with asthma. J Paediatr Child Health. 2005;41:107–111. , .
- American Academy of Pediatrics Subcommittee on Diagnosis and Management of Bronchiolitis. Diagnosis and management of bronchiolitis. Pediatrics. 2006;118:1774–1793.
- Evaluation of the utility of radiography in acute bronchiolitis. J Pediatr. 2007;150:429–433. , , , et al.
- Incidence and predisposing factors for severe disease in previously healthy term infants experiencing their first episode of bronchiolitis. Acta Paediatr. 2011;100:e17–e23. , , , et al.
- A cost effectiveness analysis of omitting radiography in diagnosis of acute bronchiolitis. Pediatr Pulmonol. 2009;44:122–127. , , , et al.
- Decreasing unnecessary utilization in acute bronchiolitis care: results from the value in inpatient pediatrics network. J Hosp Med. 2013;8:25–30. , , , et al.
- Efficacy of bronchodilator therapy in bronchiolitis. A meta‐analysis. Arch Pediatr Adolesc Med. 1996;150:1166–1172. , , , .
- Efficacy of beta2‐agonists in bronchiolitis: a reappraisal and meta‐analysis. Pediatrics. 1997;100:233–239. , .
- Bronchodilators for bronchiolitis. Cochrane Database Syst Rev. 2010;(12):CD001266. , .
- Epinephrine for bronchiolitis. Cochrane Database Syst Rev. 2011;(6):CD003123. , , , et al.
- Epinephrine and dexamethasone in children with bronchiolitis. N Engl J Med. 2009;360:2079–2089. , , , et al.
- A multicenter, randomized, double‐blind, controlled trial of nebulized epinephrine in infants with acute bronchiolitis. N Engl J Med. 2003;349:27–35. , , , et al.
- A randomized, controlled trial of the effectiveness of nebulized therapy with epinephrine compared with albuterol and saline in infants hospitalized for acute viral bronchiolitis. J Pediatr. 2002;141:818–824. , , , .
- Oral prednisolone for preschool children with acute virus‐induced wheezing. N Engl J Med. 2009;360:329–338. , , , et al.
- Glucocorticoids for acute viral bronchiolitis in infants and young children. Cochrane Database Syst Rev. 2010;(10):CD004878. , , , et al.
- Systemic corticosteroids in infant bronchiolitis: a meta‐analysis. Pediatrics. 2000;105:E44. , , , , .
- Controlled trial of oral prednisone in the emergency department treatment of children with acute asthma. Pediatrics. 1993;92:513–518. , , , .
- Methylprednisolone therapy for acute asthma in infants and toddlers: a controlled clinical trial. Pediatrics. 1990;86:350–356. , , .
- Effect of a single oral dose of prednisolone in acute childhood asthma. Lancet. 1987;1:879–882. , , , , .
- The clinical management of preterm infants with bronchiolitis. Hosp Pediatr. 2013;3:244–250. , , , , .
- Glucocorticoids and growth in asthmatic children. Pediatr Allergy Immunol. 1995;6:145–154. , .
- Adjunct corticosteroids in children hospitalized with community‐acquired pneumonia. Pediatrics. 2011;127:e255–e263. , , , , , .
- Pediatric gastroesophageal reflux disease and acid‐related conditions: trends in incidence of diagnosis and acid suppression therapy. J Med Econ. 2009;12:348–355. , , , , , .
- Healthcare costs of GERD and acid‐related conditions in pediatric patients, with comparison between histamine‐2 receptor antagonists and proton pump inhibitors. Curr Med Res Opin. 2009;25:2703–2709. , , , , , .
- Are we overprescribing antireflux medications for infants with regurgitation? Pediatrics. 2007;120:946–949. , , , .
- Proton pump inhibitor utilization patterns in infants. J Pediatr Gastroenterol Nutr. 2007;45:421–427. , , , , .
- Efficacy of proton‐pump inhibitors in children with gastroesophageal reflux disease: a systematic review. Pediatrics. 2011;127:925–935. , , , , , .
- Effectiveness and safety of proton pump inhibitors in infantile gastroesophageal reflux disease. Ann Pharmacother. 2010;44:572–576. .
- Are there risks associated with empric acid suppression treatment of infants and children suspected of having gastroesophageal reflux disease? Hosp Pediatr. 2013;3:16–23. .
- Bronchiolitis management preferences and the influence of pulse oximetry and respiratory rate on the decision to admit. Pediatrics. 2003;111:e45–e51. , , , .
- Impact of pulse oximetry and oxygen therapy on length of stay in bronchiolitis hospitalizations. Arch Pediatr Adolesc Med. 2004;158:527–530. , , , .
- Effect of oxygen supplementation on length of stay for infants hospitalized with acute viral bronchiolitis. Pediatrics. 2008;121:470–475. , .
- Oxygen therapy for bronchiolitis. Pediatrics. 2007;120:686–687; author reply 687–688. .
- Bronchiolitis‐associated hospitalizations among US children, 1980–1996. JAMA. 1999;282:1440–1446. , , , , , .
- Bronchiolitis: recent evidence on diagnosis and management. Pediatrics. 2010;125:342–349. , .
- Bronchiolitis‐associated mortality and estimates of respiratory syncytial virus‐associated deaths among US children, 1979–1997. J Infect Dis. 2001;183:16–22. , , , , .
- Observational study of two oxygen saturation targets for discharge in bronchiolitis. Arch Dis Child. 2012;97:361–363. , .
- Longitudinal assessment of hemoglobin oxygen saturation in preterm and term infants in the first six months of life. J Pediatr. 2011;159:377–383.e1. , , , et al.
- The impact of severe asthma on schoolchildren. J Asthma. 1999;36:409–417. , .
- Multi‐center, randomized trial of pulse oximetry monitoring strategies for children hospitalized for bronchiolitis. Abstract presented at: ID Week 2012; October 2012; San Diego, CA. , .
- The appropriateness method has acceptable reliability and validity for assessing overuse and underuse of surgical procedures. J Clin Epidemiol. 2012;65:1133–1143. , , , .
- Agency for Healthcare Research and Quality. HCUPnet. Kids inpatient database 2009. Available at: http://hcupnet.ahrq.gov. Accessed November 6, 2012.
- Too little? Too much? Primary care physicians' views on US health care: a brief report. Arch Intern Med. 2011;171:1582–1585. , , .
- How to implement change in clinical practice. Paediatr Respir Rev. 2003;4:340–346. .
Copyright © 2013 Society of Hospital Medicine