User login
Make no bones about it!
Make no bones about it!
We just celebrated 45 years since the passing of Title IX, which opened the floodgates for women’s participation in sports. According to a report by the National Collegiate Athletic Association,1 in this interval, the participation rate of high school girls increased 1,000 percent, and Division I colleges have the highest female athletic participation rate, with women accounting for 46.7% of athletes.
Participating in competitive sports is especially important for women because it increases self-confidence. Indeed, there is a direct relationship between athletics and women in leadership roles. A 2013 Ernst and Young survey of 821 high-level executives demonstrated that 90% of the women and 96% of the women in chief executive positions had played sports.2
Twenty years after Title IX was passed, physicians identified a triad of symptoms commonly seen in female athletes. The original definition of the female athletic triad consisted of eating disorders, irregular menstrual cycles, and reduced bone mineral density (BMD).3 Malnutrition led to abnormalities in the menstrual cycle, which in turn affected bone density. The triad was thought to most commonly affect women participating in weight-dependent or judging sports, such as gymnastics, ice-skating, and endurance running. However, many athletes remained undiagnosed because specific criteria for the triad diagnosis remained elusive.
In 2007, the American College of Sports Medicine updated the diagnostic guidelines, defining the female athlete triad as a constellation of abnormalities in energy availability, menstrual function, and BMD.4 Each of these 3 components is part of a spectrum ranging from normal to varying degrees of pathology. Thus, the female athlete no longer needs to demonstrate pathology in all 3 components of the triad to be diagnosed with the syndrome. The presence of 1 or 2 of the components on the pathologic side of the spectrum falls under the umbrella of the triad and may meet the criteria for diagnosis, prompting further assessment.
In 2014, the International Olympic Committee (IOC) coined the term relative energy deficiency in sport (RED-S).5 The IOC authors intended for RED-S to be a more comprehensive and broader definition for the triad. As defined in the IOC consensus statement, RED-S is “impaired physiological function including, but not limited to, metabolic rate, menstrual function, bone health, immunity, protein synthesis, [and] cardiovascular health caused by relative energy deficiency.”5 The statement indicates that the underlying problem is “energy deficiency relative to the balance between dietary energy intake and energy expenditure required for health and activities of daily living, growth, and sporting activities.”5 The IOC consensus statement also expands the vulnerable population, discussing the susceptibility of male athletes, athletes of nonwhite ethnicity, and athletes with a disability.
Although there is some contention as to how we should refer to this syndrome, the most important facet is that it can be identified in an office setting. Awareness is key to prevention. Unfortunately, in a 2015 survey of 931 physicians, only 37% could identify the 3 components of the triad.6 If you do not ask your patients about their nutrition, eating habits, and menstrual cycle, it is not possible to identify any potential problems.
WHY IS THIS SO IMPORTANT?
Simply stated, it is important to diagnose and treat the triad syndrome to maintain optimal bone health. About 90% of peak bone mass is acquired by age 18. Bone density continues to build until about the age of 25, after which it is only possible to maintain. If young athletes are losing bone density, it cannot be replaced. Athletes who have a relative energy deficiency and are not maximizing bone growth will potentially struggle with lower bone mineral density later in life. Early awareness of bone health is paramount to sustaining it as we age.
Osteoporosis, often called a silent disease, affects more than 75 million men and women in the United States, Europe, and Japan.7 According to the International Osteoporosis Foundation, more than 8.9 million fractures are secondary to osteoporosis worldwide each year. Astoundingly, this epidemic equates to an osteoporotic fracture every 3 seconds.8
As physicians, we need to do what we can to prevent this, and the easiest prevention is maintaining bone health and adequate nutrition early in life. We know that weight-bearing exercise is important for bone health, but it is counterintuitive to think that active patients who are running and playing sports may be negatively affecting bone health if they have a relative energy deficiency.
Many females (professional athletes, competitive athletes, or just women who want to stay active) exercise excessively and restrict calories to lose or maintain weight. This can be a formula for disaster resulting in a sidelining bone stress injury. A stress fracture occurs when bone experiences more stress or impact than it can handle from overtraining or increasing training too quickly, not providing enough time for the bone to strengthen. Stress fractures can also occur when bone mineral density declines, lowering the impact threshold. This is most often a direct result of a relative energy deficiency or poor nutrition. The incidence of stress fractures in female athletes is 2 to 3 times higher than that in male athletes, and female athletes with missed menstrual cycles have a 2 to 4 times higher risk of stress fractures than females with a normal monthly menstrual cycle.9
The triad syndrome is seen not only in women but in all athletes—including men and Paralympic athletes.10,11 According to Tenforde et al,10 male athletes, particularly those who participate in sports requiring leanness such as cycling and running, can exhibit nutritional deficits, reduced sex hormone levels, and impaired bone health.10
If we can educate patients on the importance of maintaining a healthy diet and supplying active bodies with the energy they need, then many of these injuries could be prevented.
Make no bones about it.
- Wilson AS; NCAA Research Department. 45 years of Title IX: the status of women in intercollegiate athletics. www.ncaa.org/sites/default/files/TitleIX45-295-FINAL_WEB.pdf. Accessed February 5, 2018.
- Ernst and Young; Women Athletes Business Network. Perspectives on sport and teams. www.ey.com/br/pt/about-us/our-sponsorships-and-programs/women-athletes-global-leadership-network---perspectives-on-sport-and-teams. Accessed February 5, 2018.
- Matzkin E, Curry EJ, Whitlock K. Female athlete triad: past, present, and future. J Am Acad Orthop Surg 2015; 23:424–432. doi:10.5435/JAAOS-D-14-00168
- Nattiv A, Loucks AB, Manore MM, Sanborn CF, Sundgot-Borgen J, Warren MP; American College of Sports Medicine. American College of Sports Medicine position stand. The female athlete triad. Med Sci Sports Exerc 2007; 39:1867–1882. doi:10.1249/mss.0b013e318149f111
- Mountjoy M, Sundgot-Borgen J, Burke L, et al. The IOC consensus statement: beyond the female athlete triad—relative energy deficiency in sport (RED-S). Br J Sports Med 2014; 48:491–497. doi:10.1136/bjsports-2014-093502
- Curry EJ, Logan C, Ackerman K, McInnis KC, Matzkin EG. Female athlete triad awareness among multispecialty physicians. Sports Med Open 2015; 1:38. doi:10.1186/s40798-015-0037-5
- Who are candidates for prevention and treatment for osteoporosis? Osteoporos Int 1997; 7:1–6.
- Johnell O, Kanis JA. An estimate of the worldwide prevalence and disability associated with osteoporotic fractures. Osteoporos Int 2006; 17:1726–1733. doi:10.1007/s00198-006-0172-4
- Wentz L, Liu PY, Haymes E, Ilich JZ. Females have a greater incidence of stress fractures than males in both military and athletic populations: a systemic review. Mil Med 2011; 176:420–430.
- Tenforde AS, Barrack MT, Nattiv A, Fredericson M. Parallels with the female athlete triad in male athletes. Sports Med 2016; 46:171–182. doi:10.1007/s40279-015-0411-y
- Blauwet CA, Brook EM, Tenforde AS, et al. Low energy availability, menstrual dysfunction, and low bone mineral density in individuals with a disability: implications for the para athlete population. Sports Med 2017; 47(9)1697–1708. doi:10.1007/s40279-017-0696-0
Make no bones about it!
We just celebrated 45 years since the passing of Title IX, which opened the floodgates for women’s participation in sports. According to a report by the National Collegiate Athletic Association,1 in this interval, the participation rate of high school girls increased 1,000 percent, and Division I colleges have the highest female athletic participation rate, with women accounting for 46.7% of athletes.
Participating in competitive sports is especially important for women because it increases self-confidence. Indeed, there is a direct relationship between athletics and women in leadership roles. A 2013 Ernst and Young survey of 821 high-level executives demonstrated that 90% of the women and 96% of the women in chief executive positions had played sports.2
Twenty years after Title IX was passed, physicians identified a triad of symptoms commonly seen in female athletes. The original definition of the female athletic triad consisted of eating disorders, irregular menstrual cycles, and reduced bone mineral density (BMD).3 Malnutrition led to abnormalities in the menstrual cycle, which in turn affected bone density. The triad was thought to most commonly affect women participating in weight-dependent or judging sports, such as gymnastics, ice-skating, and endurance running. However, many athletes remained undiagnosed because specific criteria for the triad diagnosis remained elusive.
In 2007, the American College of Sports Medicine updated the diagnostic guidelines, defining the female athlete triad as a constellation of abnormalities in energy availability, menstrual function, and BMD.4 Each of these 3 components is part of a spectrum ranging from normal to varying degrees of pathology. Thus, the female athlete no longer needs to demonstrate pathology in all 3 components of the triad to be diagnosed with the syndrome. The presence of 1 or 2 of the components on the pathologic side of the spectrum falls under the umbrella of the triad and may meet the criteria for diagnosis, prompting further assessment.
In 2014, the International Olympic Committee (IOC) coined the term relative energy deficiency in sport (RED-S).5 The IOC authors intended for RED-S to be a more comprehensive and broader definition for the triad. As defined in the IOC consensus statement, RED-S is “impaired physiological function including, but not limited to, metabolic rate, menstrual function, bone health, immunity, protein synthesis, [and] cardiovascular health caused by relative energy deficiency.”5 The statement indicates that the underlying problem is “energy deficiency relative to the balance between dietary energy intake and energy expenditure required for health and activities of daily living, growth, and sporting activities.”5 The IOC consensus statement also expands the vulnerable population, discussing the susceptibility of male athletes, athletes of nonwhite ethnicity, and athletes with a disability.
Although there is some contention as to how we should refer to this syndrome, the most important facet is that it can be identified in an office setting. Awareness is key to prevention. Unfortunately, in a 2015 survey of 931 physicians, only 37% could identify the 3 components of the triad.6 If you do not ask your patients about their nutrition, eating habits, and menstrual cycle, it is not possible to identify any potential problems.
WHY IS THIS SO IMPORTANT?
Simply stated, it is important to diagnose and treat the triad syndrome to maintain optimal bone health. About 90% of peak bone mass is acquired by age 18. Bone density continues to build until about the age of 25, after which it is only possible to maintain. If young athletes are losing bone density, it cannot be replaced. Athletes who have a relative energy deficiency and are not maximizing bone growth will potentially struggle with lower bone mineral density later in life. Early awareness of bone health is paramount to sustaining it as we age.
Osteoporosis, often called a silent disease, affects more than 75 million men and women in the United States, Europe, and Japan.7 According to the International Osteoporosis Foundation, more than 8.9 million fractures are secondary to osteoporosis worldwide each year. Astoundingly, this epidemic equates to an osteoporotic fracture every 3 seconds.8
As physicians, we need to do what we can to prevent this, and the easiest prevention is maintaining bone health and adequate nutrition early in life. We know that weight-bearing exercise is important for bone health, but it is counterintuitive to think that active patients who are running and playing sports may be negatively affecting bone health if they have a relative energy deficiency.
Many females (professional athletes, competitive athletes, or just women who want to stay active) exercise excessively and restrict calories to lose or maintain weight. This can be a formula for disaster resulting in a sidelining bone stress injury. A stress fracture occurs when bone experiences more stress or impact than it can handle from overtraining or increasing training too quickly, not providing enough time for the bone to strengthen. Stress fractures can also occur when bone mineral density declines, lowering the impact threshold. This is most often a direct result of a relative energy deficiency or poor nutrition. The incidence of stress fractures in female athletes is 2 to 3 times higher than that in male athletes, and female athletes with missed menstrual cycles have a 2 to 4 times higher risk of stress fractures than females with a normal monthly menstrual cycle.9
The triad syndrome is seen not only in women but in all athletes—including men and Paralympic athletes.10,11 According to Tenforde et al,10 male athletes, particularly those who participate in sports requiring leanness such as cycling and running, can exhibit nutritional deficits, reduced sex hormone levels, and impaired bone health.10
If we can educate patients on the importance of maintaining a healthy diet and supplying active bodies with the energy they need, then many of these injuries could be prevented.
Make no bones about it.
Make no bones about it!
We just celebrated 45 years since the passing of Title IX, which opened the floodgates for women’s participation in sports. According to a report by the National Collegiate Athletic Association,1 in this interval, the participation rate of high school girls increased 1,000 percent, and Division I colleges have the highest female athletic participation rate, with women accounting for 46.7% of athletes.
Participating in competitive sports is especially important for women because it increases self-confidence. Indeed, there is a direct relationship between athletics and women in leadership roles. A 2013 Ernst and Young survey of 821 high-level executives demonstrated that 90% of the women and 96% of the women in chief executive positions had played sports.2
Twenty years after Title IX was passed, physicians identified a triad of symptoms commonly seen in female athletes. The original definition of the female athletic triad consisted of eating disorders, irregular menstrual cycles, and reduced bone mineral density (BMD).3 Malnutrition led to abnormalities in the menstrual cycle, which in turn affected bone density. The triad was thought to most commonly affect women participating in weight-dependent or judging sports, such as gymnastics, ice-skating, and endurance running. However, many athletes remained undiagnosed because specific criteria for the triad diagnosis remained elusive.
In 2007, the American College of Sports Medicine updated the diagnostic guidelines, defining the female athlete triad as a constellation of abnormalities in energy availability, menstrual function, and BMD.4 Each of these 3 components is part of a spectrum ranging from normal to varying degrees of pathology. Thus, the female athlete no longer needs to demonstrate pathology in all 3 components of the triad to be diagnosed with the syndrome. The presence of 1 or 2 of the components on the pathologic side of the spectrum falls under the umbrella of the triad and may meet the criteria for diagnosis, prompting further assessment.
In 2014, the International Olympic Committee (IOC) coined the term relative energy deficiency in sport (RED-S).5 The IOC authors intended for RED-S to be a more comprehensive and broader definition for the triad. As defined in the IOC consensus statement, RED-S is “impaired physiological function including, but not limited to, metabolic rate, menstrual function, bone health, immunity, protein synthesis, [and] cardiovascular health caused by relative energy deficiency.”5 The statement indicates that the underlying problem is “energy deficiency relative to the balance between dietary energy intake and energy expenditure required for health and activities of daily living, growth, and sporting activities.”5 The IOC consensus statement also expands the vulnerable population, discussing the susceptibility of male athletes, athletes of nonwhite ethnicity, and athletes with a disability.
Although there is some contention as to how we should refer to this syndrome, the most important facet is that it can be identified in an office setting. Awareness is key to prevention. Unfortunately, in a 2015 survey of 931 physicians, only 37% could identify the 3 components of the triad.6 If you do not ask your patients about their nutrition, eating habits, and menstrual cycle, it is not possible to identify any potential problems.
WHY IS THIS SO IMPORTANT?
Simply stated, it is important to diagnose and treat the triad syndrome to maintain optimal bone health. About 90% of peak bone mass is acquired by age 18. Bone density continues to build until about the age of 25, after which it is only possible to maintain. If young athletes are losing bone density, it cannot be replaced. Athletes who have a relative energy deficiency and are not maximizing bone growth will potentially struggle with lower bone mineral density later in life. Early awareness of bone health is paramount to sustaining it as we age.
Osteoporosis, often called a silent disease, affects more than 75 million men and women in the United States, Europe, and Japan.7 According to the International Osteoporosis Foundation, more than 8.9 million fractures are secondary to osteoporosis worldwide each year. Astoundingly, this epidemic equates to an osteoporotic fracture every 3 seconds.8
As physicians, we need to do what we can to prevent this, and the easiest prevention is maintaining bone health and adequate nutrition early in life. We know that weight-bearing exercise is important for bone health, but it is counterintuitive to think that active patients who are running and playing sports may be negatively affecting bone health if they have a relative energy deficiency.
Many females (professional athletes, competitive athletes, or just women who want to stay active) exercise excessively and restrict calories to lose or maintain weight. This can be a formula for disaster resulting in a sidelining bone stress injury. A stress fracture occurs when bone experiences more stress or impact than it can handle from overtraining or increasing training too quickly, not providing enough time for the bone to strengthen. Stress fractures can also occur when bone mineral density declines, lowering the impact threshold. This is most often a direct result of a relative energy deficiency or poor nutrition. The incidence of stress fractures in female athletes is 2 to 3 times higher than that in male athletes, and female athletes with missed menstrual cycles have a 2 to 4 times higher risk of stress fractures than females with a normal monthly menstrual cycle.9
The triad syndrome is seen not only in women but in all athletes—including men and Paralympic athletes.10,11 According to Tenforde et al,10 male athletes, particularly those who participate in sports requiring leanness such as cycling and running, can exhibit nutritional deficits, reduced sex hormone levels, and impaired bone health.10
If we can educate patients on the importance of maintaining a healthy diet and supplying active bodies with the energy they need, then many of these injuries could be prevented.
Make no bones about it.
- Wilson AS; NCAA Research Department. 45 years of Title IX: the status of women in intercollegiate athletics. www.ncaa.org/sites/default/files/TitleIX45-295-FINAL_WEB.pdf. Accessed February 5, 2018.
- Ernst and Young; Women Athletes Business Network. Perspectives on sport and teams. www.ey.com/br/pt/about-us/our-sponsorships-and-programs/women-athletes-global-leadership-network---perspectives-on-sport-and-teams. Accessed February 5, 2018.
- Matzkin E, Curry EJ, Whitlock K. Female athlete triad: past, present, and future. J Am Acad Orthop Surg 2015; 23:424–432. doi:10.5435/JAAOS-D-14-00168
- Nattiv A, Loucks AB, Manore MM, Sanborn CF, Sundgot-Borgen J, Warren MP; American College of Sports Medicine. American College of Sports Medicine position stand. The female athlete triad. Med Sci Sports Exerc 2007; 39:1867–1882. doi:10.1249/mss.0b013e318149f111
- Mountjoy M, Sundgot-Borgen J, Burke L, et al. The IOC consensus statement: beyond the female athlete triad—relative energy deficiency in sport (RED-S). Br J Sports Med 2014; 48:491–497. doi:10.1136/bjsports-2014-093502
- Curry EJ, Logan C, Ackerman K, McInnis KC, Matzkin EG. Female athlete triad awareness among multispecialty physicians. Sports Med Open 2015; 1:38. doi:10.1186/s40798-015-0037-5
- Who are candidates for prevention and treatment for osteoporosis? Osteoporos Int 1997; 7:1–6.
- Johnell O, Kanis JA. An estimate of the worldwide prevalence and disability associated with osteoporotic fractures. Osteoporos Int 2006; 17:1726–1733. doi:10.1007/s00198-006-0172-4
- Wentz L, Liu PY, Haymes E, Ilich JZ. Females have a greater incidence of stress fractures than males in both military and athletic populations: a systemic review. Mil Med 2011; 176:420–430.
- Tenforde AS, Barrack MT, Nattiv A, Fredericson M. Parallels with the female athlete triad in male athletes. Sports Med 2016; 46:171–182. doi:10.1007/s40279-015-0411-y
- Blauwet CA, Brook EM, Tenforde AS, et al. Low energy availability, menstrual dysfunction, and low bone mineral density in individuals with a disability: implications for the para athlete population. Sports Med 2017; 47(9)1697–1708. doi:10.1007/s40279-017-0696-0
- Wilson AS; NCAA Research Department. 45 years of Title IX: the status of women in intercollegiate athletics. www.ncaa.org/sites/default/files/TitleIX45-295-FINAL_WEB.pdf. Accessed February 5, 2018.
- Ernst and Young; Women Athletes Business Network. Perspectives on sport and teams. www.ey.com/br/pt/about-us/our-sponsorships-and-programs/women-athletes-global-leadership-network---perspectives-on-sport-and-teams. Accessed February 5, 2018.
- Matzkin E, Curry EJ, Whitlock K. Female athlete triad: past, present, and future. J Am Acad Orthop Surg 2015; 23:424–432. doi:10.5435/JAAOS-D-14-00168
- Nattiv A, Loucks AB, Manore MM, Sanborn CF, Sundgot-Borgen J, Warren MP; American College of Sports Medicine. American College of Sports Medicine position stand. The female athlete triad. Med Sci Sports Exerc 2007; 39:1867–1882. doi:10.1249/mss.0b013e318149f111
- Mountjoy M, Sundgot-Borgen J, Burke L, et al. The IOC consensus statement: beyond the female athlete triad—relative energy deficiency in sport (RED-S). Br J Sports Med 2014; 48:491–497. doi:10.1136/bjsports-2014-093502
- Curry EJ, Logan C, Ackerman K, McInnis KC, Matzkin EG. Female athlete triad awareness among multispecialty physicians. Sports Med Open 2015; 1:38. doi:10.1186/s40798-015-0037-5
- Who are candidates for prevention and treatment for osteoporosis? Osteoporos Int 1997; 7:1–6.
- Johnell O, Kanis JA. An estimate of the worldwide prevalence and disability associated with osteoporotic fractures. Osteoporos Int 2006; 17:1726–1733. doi:10.1007/s00198-006-0172-4
- Wentz L, Liu PY, Haymes E, Ilich JZ. Females have a greater incidence of stress fractures than males in both military and athletic populations: a systemic review. Mil Med 2011; 176:420–430.
- Tenforde AS, Barrack MT, Nattiv A, Fredericson M. Parallels with the female athlete triad in male athletes. Sports Med 2016; 46:171–182. doi:10.1007/s40279-015-0411-y
- Blauwet CA, Brook EM, Tenforde AS, et al. Low energy availability, menstrual dysfunction, and low bone mineral density in individuals with a disability: implications for the para athlete population. Sports Med 2017; 47(9)1697–1708. doi:10.1007/s40279-017-0696-0
Musculoskeletal ultrasonography has arrived
A 50-year-old woman with hypertension presents with a history of polyarticular small-joint pain for the last 3 months. Her pain is worse in the morning, and it affects her metacarpal, proximal, and distal phalangeal joints. She describes intermittent swelling of her hands and morning stiffness lasting 15 to 30 minutes.
Her physical examination is unremarkable, with no evidence of active inflammation (synovitis), joint tenderness, restrictions in movement, or deformity. Her description of her symptoms raises suspicion for an inflammatory arthritis, but her physical examination does not support this diagnosis.
Bedside musculoskeletal ultrasonography of her wrists reveals synovial hypertrophy, and power Doppler shows active inflammation, findings consistent with synovitis (Figure 1).
This scenario illustrates how musculoskeletal ultrasonography can prevent delayed diagnosis, thus directing the ordering of appropriate laboratory studies and allowing treatment for pain relief to be started promptly.
ULTRASONOGRAPHY HAS GAINED A SOLID ROLE
Ultrasonography has gained a solid role in the care of patients with musculoskeletal conditions.
Using obtained images, as well as power Doppler to assess inflammation, the clinician can visualize superficial anatomic structures, including the skin, muscles, joints, nerves, and the cortical layer of bone. Combining the dynamic assessment with the clinical history and findings of the physical examination makes musculoskeletal ultrasonography a powerful tool for diagnosis and management.1
In this issue, Forney and Delzell2 review the clinical use of ultrasonography of the muscles and bones and its advantages and disadvantages compared with other imaging methods. They describe its gain in popularity over the last decade and its incorporation into clinical care in multiple medical subspecialties.
Musculoskeletal ultrasonography is performed and interpreted by specially trained sonographers. It should be viewed as a complementary procedure, not as a replacement for a thorough and systematic clinical examination.3
ADVANTAGES ARE MANY
A major advantage of musculoskeletal ultrasonography over other imaging techniques is its capacity to dynamically assess joint and tendon movements4 and to immediately interpret them in real time.
In rheumatology, where it has made the biggest impact, it can help evaluate inflammatory and noninflammatory rheumatic diseases, assess treatment response, and guide joint injections.1 It has been demonstrated to significantly improve timely diagnosis and management,5 decrease dependence on other imaging modalities, and reduce healthcare costs.6
With its easy portability, ultrasonography has also been integrated into orthopedics, podiatry, physical medicine and rehabilitation, sports medicine, and emergency medicine. Its role is expanding to include the assessment of the skin in systemic sclerosis, parotid and submandibular glands in Sjögren syndrome, nails in patients with psoriasis, and temporal arteries in giant cell arteritis.
A ROLE IN MEDICAL EDUCATION
Musculoskeletal ultrasonography has entered into medical education, with an increasing number of medical schools incorporating it into their curriculum over the last few years.7 It enhances student learning of anatomy, the physical examination, and pathologic findings of rheumatic diseases.7,8 Some internal medicine residency programs have added ultrasonography to help identify anatomic structures for invasive procedures, increasing patient safety and reducing procedural complications.9
It has been incorporated into the core curriculum in many rheumatology fellowship training programs.10 Rheumatologists can now also take additional courses to enhance their skills and become certified sonographers.
Musculoskeletal ultrasonography has proven to be a useful adjunct to the physical examination. With its many advantages, it has gained acceptance and is now a mainstay in many subspecialties.
- Cannella AC, Kissin EY, Torralba KD, Higgs JB, Kaeley GS. Evolution of musculoskeletal ultrasound in the United States: implementation and practice in rheumatology. Arthritis Care Res (Hoboken) 2014; 66(1):7–13. doi:10.1002/acr.22183
- Forney MC, Delzell PB. Musculoskeletal ultrasonography basics. Cleve Clin J Med 2018; 85(4):283–300. doi:10.3949/ccjm.85a.17014
- McAlindon T, Kissin E, Nazarian L, et al. American College of Rheumatology report on reasonable use of musculoskeletal ultrasonography in rheumatology clinical practice. Arthritis Care Res (Hoboken) 2012; 64(11):1625–1640. doi:10.1002/acr.21836
- Backhaus M, Burmester GR, Gerber T, et al; Working Group for Musculoskeletal Ultrasound in the EULAR Standing Committee on International Clinical Studies including Therapeutic Trials. Guidelines for musculoskeletal ultrasound in rheumatology. Ann Rheum Dis 2001; 60(7):641–649.
- Micu MC, Alcalde M, Saenz JI, et al. Impact of musculoskeletal ultrasound in an outpatient rheumatology clinic. Arthritis Care Res (Hoboken) 2013; 65(4):615–621. doi:10.1002/acr.21853
- Kay JC, Higgs JB, Battafarano DF. Utility of musculoskeletal ultrasound in a Department of Defense rheumatology practice: a four-year retrospective experience. Arthritis Care Res (Hoboken) 2014; 66(1):14–18. doi:10.1002/acr.22127
- Dinh VA, Fu JY, Lu S, Chiem A, Fox JC, Blaivas M. Integration of ultrasound in medical education at United States medical schools. J Ultrasound Med 2016; 35(2):413–419. doi:10.7863/ultra.15.05073
- Wright SA, Bell AL. Enhancement of undergraduate rheumatology teaching through the use of musculoskeletal ultrasound. Rheumatology (Oxford) 2008; 47(10):1564–1566. doi:10.1093/rheumatology/ken324
- Keddis MT, Cullen MW, Reed DA, et al. Effectiveness of an ultrasound training module for internal medicine residents. BMC Med Educ 2011; 11:75. doi:0.1186/1472-6920-11-75
- Torralba K, Cannella AC, Kissin EY, et al. Musculoskeletal ultrasound instruction in adult rheumatology fellowship programs. Arthritis Care Res (Hoboken) 2017. Epub ahead of print. doi:10.1002/acr.23336
A 50-year-old woman with hypertension presents with a history of polyarticular small-joint pain for the last 3 months. Her pain is worse in the morning, and it affects her metacarpal, proximal, and distal phalangeal joints. She describes intermittent swelling of her hands and morning stiffness lasting 15 to 30 minutes.
Her physical examination is unremarkable, with no evidence of active inflammation (synovitis), joint tenderness, restrictions in movement, or deformity. Her description of her symptoms raises suspicion for an inflammatory arthritis, but her physical examination does not support this diagnosis.
Bedside musculoskeletal ultrasonography of her wrists reveals synovial hypertrophy, and power Doppler shows active inflammation, findings consistent with synovitis (Figure 1).
This scenario illustrates how musculoskeletal ultrasonography can prevent delayed diagnosis, thus directing the ordering of appropriate laboratory studies and allowing treatment for pain relief to be started promptly.
ULTRASONOGRAPHY HAS GAINED A SOLID ROLE
Ultrasonography has gained a solid role in the care of patients with musculoskeletal conditions.
Using obtained images, as well as power Doppler to assess inflammation, the clinician can visualize superficial anatomic structures, including the skin, muscles, joints, nerves, and the cortical layer of bone. Combining the dynamic assessment with the clinical history and findings of the physical examination makes musculoskeletal ultrasonography a powerful tool for diagnosis and management.1
In this issue, Forney and Delzell2 review the clinical use of ultrasonography of the muscles and bones and its advantages and disadvantages compared with other imaging methods. They describe its gain in popularity over the last decade and its incorporation into clinical care in multiple medical subspecialties.
Musculoskeletal ultrasonography is performed and interpreted by specially trained sonographers. It should be viewed as a complementary procedure, not as a replacement for a thorough and systematic clinical examination.3
ADVANTAGES ARE MANY
A major advantage of musculoskeletal ultrasonography over other imaging techniques is its capacity to dynamically assess joint and tendon movements4 and to immediately interpret them in real time.
In rheumatology, where it has made the biggest impact, it can help evaluate inflammatory and noninflammatory rheumatic diseases, assess treatment response, and guide joint injections.1 It has been demonstrated to significantly improve timely diagnosis and management,5 decrease dependence on other imaging modalities, and reduce healthcare costs.6
With its easy portability, ultrasonography has also been integrated into orthopedics, podiatry, physical medicine and rehabilitation, sports medicine, and emergency medicine. Its role is expanding to include the assessment of the skin in systemic sclerosis, parotid and submandibular glands in Sjögren syndrome, nails in patients with psoriasis, and temporal arteries in giant cell arteritis.
A ROLE IN MEDICAL EDUCATION
Musculoskeletal ultrasonography has entered into medical education, with an increasing number of medical schools incorporating it into their curriculum over the last few years.7 It enhances student learning of anatomy, the physical examination, and pathologic findings of rheumatic diseases.7,8 Some internal medicine residency programs have added ultrasonography to help identify anatomic structures for invasive procedures, increasing patient safety and reducing procedural complications.9
It has been incorporated into the core curriculum in many rheumatology fellowship training programs.10 Rheumatologists can now also take additional courses to enhance their skills and become certified sonographers.
Musculoskeletal ultrasonography has proven to be a useful adjunct to the physical examination. With its many advantages, it has gained acceptance and is now a mainstay in many subspecialties.
A 50-year-old woman with hypertension presents with a history of polyarticular small-joint pain for the last 3 months. Her pain is worse in the morning, and it affects her metacarpal, proximal, and distal phalangeal joints. She describes intermittent swelling of her hands and morning stiffness lasting 15 to 30 minutes.
Her physical examination is unremarkable, with no evidence of active inflammation (synovitis), joint tenderness, restrictions in movement, or deformity. Her description of her symptoms raises suspicion for an inflammatory arthritis, but her physical examination does not support this diagnosis.
Bedside musculoskeletal ultrasonography of her wrists reveals synovial hypertrophy, and power Doppler shows active inflammation, findings consistent with synovitis (Figure 1).
This scenario illustrates how musculoskeletal ultrasonography can prevent delayed diagnosis, thus directing the ordering of appropriate laboratory studies and allowing treatment for pain relief to be started promptly.
ULTRASONOGRAPHY HAS GAINED A SOLID ROLE
Ultrasonography has gained a solid role in the care of patients with musculoskeletal conditions.
Using obtained images, as well as power Doppler to assess inflammation, the clinician can visualize superficial anatomic structures, including the skin, muscles, joints, nerves, and the cortical layer of bone. Combining the dynamic assessment with the clinical history and findings of the physical examination makes musculoskeletal ultrasonography a powerful tool for diagnosis and management.1
In this issue, Forney and Delzell2 review the clinical use of ultrasonography of the muscles and bones and its advantages and disadvantages compared with other imaging methods. They describe its gain in popularity over the last decade and its incorporation into clinical care in multiple medical subspecialties.
Musculoskeletal ultrasonography is performed and interpreted by specially trained sonographers. It should be viewed as a complementary procedure, not as a replacement for a thorough and systematic clinical examination.3
ADVANTAGES ARE MANY
A major advantage of musculoskeletal ultrasonography over other imaging techniques is its capacity to dynamically assess joint and tendon movements4 and to immediately interpret them in real time.
In rheumatology, where it has made the biggest impact, it can help evaluate inflammatory and noninflammatory rheumatic diseases, assess treatment response, and guide joint injections.1 It has been demonstrated to significantly improve timely diagnosis and management,5 decrease dependence on other imaging modalities, and reduce healthcare costs.6
With its easy portability, ultrasonography has also been integrated into orthopedics, podiatry, physical medicine and rehabilitation, sports medicine, and emergency medicine. Its role is expanding to include the assessment of the skin in systemic sclerosis, parotid and submandibular glands in Sjögren syndrome, nails in patients with psoriasis, and temporal arteries in giant cell arteritis.
A ROLE IN MEDICAL EDUCATION
Musculoskeletal ultrasonography has entered into medical education, with an increasing number of medical schools incorporating it into their curriculum over the last few years.7 It enhances student learning of anatomy, the physical examination, and pathologic findings of rheumatic diseases.7,8 Some internal medicine residency programs have added ultrasonography to help identify anatomic structures for invasive procedures, increasing patient safety and reducing procedural complications.9
It has been incorporated into the core curriculum in many rheumatology fellowship training programs.10 Rheumatologists can now also take additional courses to enhance their skills and become certified sonographers.
Musculoskeletal ultrasonography has proven to be a useful adjunct to the physical examination. With its many advantages, it has gained acceptance and is now a mainstay in many subspecialties.
- Cannella AC, Kissin EY, Torralba KD, Higgs JB, Kaeley GS. Evolution of musculoskeletal ultrasound in the United States: implementation and practice in rheumatology. Arthritis Care Res (Hoboken) 2014; 66(1):7–13. doi:10.1002/acr.22183
- Forney MC, Delzell PB. Musculoskeletal ultrasonography basics. Cleve Clin J Med 2018; 85(4):283–300. doi:10.3949/ccjm.85a.17014
- McAlindon T, Kissin E, Nazarian L, et al. American College of Rheumatology report on reasonable use of musculoskeletal ultrasonography in rheumatology clinical practice. Arthritis Care Res (Hoboken) 2012; 64(11):1625–1640. doi:10.1002/acr.21836
- Backhaus M, Burmester GR, Gerber T, et al; Working Group for Musculoskeletal Ultrasound in the EULAR Standing Committee on International Clinical Studies including Therapeutic Trials. Guidelines for musculoskeletal ultrasound in rheumatology. Ann Rheum Dis 2001; 60(7):641–649.
- Micu MC, Alcalde M, Saenz JI, et al. Impact of musculoskeletal ultrasound in an outpatient rheumatology clinic. Arthritis Care Res (Hoboken) 2013; 65(4):615–621. doi:10.1002/acr.21853
- Kay JC, Higgs JB, Battafarano DF. Utility of musculoskeletal ultrasound in a Department of Defense rheumatology practice: a four-year retrospective experience. Arthritis Care Res (Hoboken) 2014; 66(1):14–18. doi:10.1002/acr.22127
- Dinh VA, Fu JY, Lu S, Chiem A, Fox JC, Blaivas M. Integration of ultrasound in medical education at United States medical schools. J Ultrasound Med 2016; 35(2):413–419. doi:10.7863/ultra.15.05073
- Wright SA, Bell AL. Enhancement of undergraduate rheumatology teaching through the use of musculoskeletal ultrasound. Rheumatology (Oxford) 2008; 47(10):1564–1566. doi:10.1093/rheumatology/ken324
- Keddis MT, Cullen MW, Reed DA, et al. Effectiveness of an ultrasound training module for internal medicine residents. BMC Med Educ 2011; 11:75. doi:0.1186/1472-6920-11-75
- Torralba K, Cannella AC, Kissin EY, et al. Musculoskeletal ultrasound instruction in adult rheumatology fellowship programs. Arthritis Care Res (Hoboken) 2017. Epub ahead of print. doi:10.1002/acr.23336
- Cannella AC, Kissin EY, Torralba KD, Higgs JB, Kaeley GS. Evolution of musculoskeletal ultrasound in the United States: implementation and practice in rheumatology. Arthritis Care Res (Hoboken) 2014; 66(1):7–13. doi:10.1002/acr.22183
- Forney MC, Delzell PB. Musculoskeletal ultrasonography basics. Cleve Clin J Med 2018; 85(4):283–300. doi:10.3949/ccjm.85a.17014
- McAlindon T, Kissin E, Nazarian L, et al. American College of Rheumatology report on reasonable use of musculoskeletal ultrasonography in rheumatology clinical practice. Arthritis Care Res (Hoboken) 2012; 64(11):1625–1640. doi:10.1002/acr.21836
- Backhaus M, Burmester GR, Gerber T, et al; Working Group for Musculoskeletal Ultrasound in the EULAR Standing Committee on International Clinical Studies including Therapeutic Trials. Guidelines for musculoskeletal ultrasound in rheumatology. Ann Rheum Dis 2001; 60(7):641–649.
- Micu MC, Alcalde M, Saenz JI, et al. Impact of musculoskeletal ultrasound in an outpatient rheumatology clinic. Arthritis Care Res (Hoboken) 2013; 65(4):615–621. doi:10.1002/acr.21853
- Kay JC, Higgs JB, Battafarano DF. Utility of musculoskeletal ultrasound in a Department of Defense rheumatology practice: a four-year retrospective experience. Arthritis Care Res (Hoboken) 2014; 66(1):14–18. doi:10.1002/acr.22127
- Dinh VA, Fu JY, Lu S, Chiem A, Fox JC, Blaivas M. Integration of ultrasound in medical education at United States medical schools. J Ultrasound Med 2016; 35(2):413–419. doi:10.7863/ultra.15.05073
- Wright SA, Bell AL. Enhancement of undergraduate rheumatology teaching through the use of musculoskeletal ultrasound. Rheumatology (Oxford) 2008; 47(10):1564–1566. doi:10.1093/rheumatology/ken324
- Keddis MT, Cullen MW, Reed DA, et al. Effectiveness of an ultrasound training module for internal medicine residents. BMC Med Educ 2011; 11:75. doi:0.1186/1472-6920-11-75
- Torralba K, Cannella AC, Kissin EY, et al. Musculoskeletal ultrasound instruction in adult rheumatology fellowship programs. Arthritis Care Res (Hoboken) 2017. Epub ahead of print. doi:10.1002/acr.23336
Reducing SNF Readmissions: At What Cost?
The landscape of postacute care in skilled nursing facilities (SNFs) in the United States is evolving. As the population ages, a growing number of elderly persons are being discharged to SNFs at an enormous cost and with clear evidence of disappointing outcomes. The reaction to these trends includes payment reforms that “bundle” hospital and postacute care, act as incentives to discourage SNFs, or penalize SNFs for undesired patient outcomes. Hospitalists are expected to increasingly feel the effect of these reforms.1
Thus, hospitals are demonstrating renewed interest in reducing readmissions from SNFs. In this issue of Journal of Hospital Medicine, Rosen and colleagues present the results of the Enhanced Care Program (ECP), a multicomponent intervention consisting of 9 nurse practitioners (NPs), a pharmacist, a pharmacy technician, a nurse educator, a program administrator, and a medical director.2 These providers are deployed to 8 SNFs around a large teaching hospital, providing direct clinical care as well as 24/7 call availability for enrolled patients, robust medication reconciliation, and monthly education for SNF nursing staff. A unique aspect of this model was that individual attending physicians in the associated SNFs could decide whether to enroll their patients in the model; patients not enrolled represented a contemporaneous control cohort. The authors found a nearly 30% reduction in the odds of 30-day readmission (OR 0.71 [0.60–0.85] after adjustment), which was robust to multiple sensitivity analyses, including a propensity-matched cohort comparison. The authors should be commended for working to mitigate these potential confounders, thereby strengthening their conclusions. Such a large reduction in readmissions reflects their high underlying prevalence (23% in the nonintervention cohort).
This report closely follows the evaluation of a similar program at the Cleveland Clinic called Connected Care Model (CCM), in which 4 physicians and 5 NPs or physician assistants provided care, including 24/7 call availability, in 7 associated SNFs.3 In a retrospective pre-post analysis comparing the 30-day readmission rates of these SNFs with those of others in the network, similar reductions in readmissions were observed. ECP and CCM represent important extensions of a much larger body of evidence, from the Evercare model4 to the Initiative to Reduce Avoidable Hospitalizations demonstration project, which suggests that adding NPs to nursing homes reduces hospitalizations.5
However, several factors have to be considered before disseminating ECP or CCM. First, other promising “proof of concept” quality improvement studies were not efficacious when rigorously tested in nursing homes.6 Second, these programs are representative of large academic medical centers, which may establish different relationships with different SNFs compared with smaller or less well-resourced hospitals. As the Initiative to Reduce Hospitalizations demonstrated, even a fundamentally similar intervention can have extremely different results depending on the nursing homes involved,5 and the science behind establishing effective hospital–SNF partnerships is still in its infancy.7 Third, both studies have significant methodological limitations, including most importantly that they are conducted within SNFs selected to be part of their hospitals’ network.
These significant early efforts also present an opportunity to reconsider the underlying assumption of these models: that adding more supervisory clinicians to SNFs is the right approach to reduce hospitalizations. Although adding resources is an attractive “plug and play” solution for many problems in healthcare delivery, placing only 1 NP in each of the 15,583 certified nursing facilities in the United States would employ fully 10% of the entire NP workforce. Amid rising concerns about costs related to our aging population, these interventions face substantial headwinds toward becoming the standard of care without demonstrating cost effectiveness. Furthermore, many SNF directors might suggest that hospitals and hospitalists working with them to address fundamental (but much more intransigent) problems in SNFs, such as high staff turnover, low concentration of highly skilled staff (RNs and MDs), regulatory burden, and hospitals using SNFs like stepdown units, could represent a generalizable and sustainable solution.
We realize that this argument is tricky for hospitalists because its underlying logic (care has become too complex, patients are too sick, and dedicated personnel are needed) also played a major role in establishing our existence. One possibility is that like hospitalists, NPs and a growing cadre of “SNFists” will become major drivers of quality improvement, education, and leadership locally at these facilities, thereby leading to sustainable change.8 Similarly, current conditions may drive recognition that a specific set of skills is required to function effectively in the SNF environment,9 just as we believe hospitalists need unique skills to excel in today’s hospital environment.
Studies such as that of Rosen et al. are valuable for JHM because they prompt us to recognize that we as hospitalists have much to share and learn from nursing homes and the dedicated practitioners who work there. In fact, we argue that few places in the healthcare system are more in need of innovation than hospital–nursing home relationships, and hospitalists do not just have a vested clinical interest; in many ways, we see a mirror of our own development as a “specialty.” We encourage hospitals and hospitalists to take up this challenge on behalf of some of the most vulnerable patients in our system during critical times in their care trajectory. As the Commission for Long-Term Care (www.ltccommission.org) wrote in its final report to Congress: “The need is great. The time to act is now.”
Disclosures
Dr. Burke is supported by a VA Health Services Research and Development (HSR&D) Career Development Award. All opinions are those of the authors and do not necessarily represent those of the Department of Veterans Affairs. Dr. Greysen has nothing to disclose.
1. Burke RE, Cumbler E, Coleman EA, Levy C. Post-acute care reform: Implications and opportunities for hospitalists. J Hosp Med. 2017;12(1):46-51. 10.3810/hp.2012.02.958. PubMed
2. Rosen BT, Halbert RJ, Hart K, Diniz MA, Isonaka S, Black JT. The enhanced care program: Impact of a care transitions program on 30-day hospital readmissions for patients discharged from an acute care facility to skilled nursing facilities. J Hosp Med. 2018;13(4):229-235.
3. Rothberg MB. Impact of a connected care model on 30-day readmission rates from skilled nursing facilities. J Hosp Med. 2017;12(4):238-244. 10.12788/jhm.2710. PubMed
4. Kane RL, Keckhafer G, Flood S, Bershadsky B, Siadaty MS. The effect of Evercare on hospital use. J Am Geriatr Soc. 2003;51(10):1427-1434. 10.1046/j.1532-5415.2003.51461.x. PubMed
5. Ingber MJ, Feng Z, Khatutsky G, et al. Initiative to reduce avoidable hospitalizations among nursing facility residents shows promising results. Health Aff Proj Hope. 2017;36(3):441-450. 10.1377/hlthaff.2016.1310. PubMed
6. Kane RL, Huckfeldt P, Tappen R, et al. Effects of an intervention to reduce hospitalizations from nursing homes: A randomized implementation trial of the INTERACT Program. JAMA Intern Med. 2017;177(9):1257-1264. 10.1001/jamainternmed.2017.2657. PubMed
7. Lage DE, Rusinak D, Carr D, Grabowski DC, Ackerly DC. Creating a network of high-quality skilled nursing facilities: preliminary data on the postacute care quality improvement experiences of an accountable care organization. J Am Geriatr Soc. 2015;63(4):804-808. 10.1111/jgs.13351. PubMed
8. Ryskina KL, Polsky D, Werner RM. Physicians and advanced practitioners specializing in nursing home care. JAMA. 2017;318(20):2040-2042. 10.1001/jama.2017.13378. PubMed
9. Gillespie SM, Levy CR, Katz PR. What exactly is an “SNF-ist?” JAMA Intern Med. 2018;178(1):153-154. 10.1001/jamainternmed.2017.7212. PubMed
The landscape of postacute care in skilled nursing facilities (SNFs) in the United States is evolving. As the population ages, a growing number of elderly persons are being discharged to SNFs at an enormous cost and with clear evidence of disappointing outcomes. The reaction to these trends includes payment reforms that “bundle” hospital and postacute care, act as incentives to discourage SNFs, or penalize SNFs for undesired patient outcomes. Hospitalists are expected to increasingly feel the effect of these reforms.1
Thus, hospitals are demonstrating renewed interest in reducing readmissions from SNFs. In this issue of Journal of Hospital Medicine, Rosen and colleagues present the results of the Enhanced Care Program (ECP), a multicomponent intervention consisting of 9 nurse practitioners (NPs), a pharmacist, a pharmacy technician, a nurse educator, a program administrator, and a medical director.2 These providers are deployed to 8 SNFs around a large teaching hospital, providing direct clinical care as well as 24/7 call availability for enrolled patients, robust medication reconciliation, and monthly education for SNF nursing staff. A unique aspect of this model was that individual attending physicians in the associated SNFs could decide whether to enroll their patients in the model; patients not enrolled represented a contemporaneous control cohort. The authors found a nearly 30% reduction in the odds of 30-day readmission (OR 0.71 [0.60–0.85] after adjustment), which was robust to multiple sensitivity analyses, including a propensity-matched cohort comparison. The authors should be commended for working to mitigate these potential confounders, thereby strengthening their conclusions. Such a large reduction in readmissions reflects their high underlying prevalence (23% in the nonintervention cohort).
This report closely follows the evaluation of a similar program at the Cleveland Clinic called Connected Care Model (CCM), in which 4 physicians and 5 NPs or physician assistants provided care, including 24/7 call availability, in 7 associated SNFs.3 In a retrospective pre-post analysis comparing the 30-day readmission rates of these SNFs with those of others in the network, similar reductions in readmissions were observed. ECP and CCM represent important extensions of a much larger body of evidence, from the Evercare model4 to the Initiative to Reduce Avoidable Hospitalizations demonstration project, which suggests that adding NPs to nursing homes reduces hospitalizations.5
However, several factors have to be considered before disseminating ECP or CCM. First, other promising “proof of concept” quality improvement studies were not efficacious when rigorously tested in nursing homes.6 Second, these programs are representative of large academic medical centers, which may establish different relationships with different SNFs compared with smaller or less well-resourced hospitals. As the Initiative to Reduce Hospitalizations demonstrated, even a fundamentally similar intervention can have extremely different results depending on the nursing homes involved,5 and the science behind establishing effective hospital–SNF partnerships is still in its infancy.7 Third, both studies have significant methodological limitations, including most importantly that they are conducted within SNFs selected to be part of their hospitals’ network.
These significant early efforts also present an opportunity to reconsider the underlying assumption of these models: that adding more supervisory clinicians to SNFs is the right approach to reduce hospitalizations. Although adding resources is an attractive “plug and play” solution for many problems in healthcare delivery, placing only 1 NP in each of the 15,583 certified nursing facilities in the United States would employ fully 10% of the entire NP workforce. Amid rising concerns about costs related to our aging population, these interventions face substantial headwinds toward becoming the standard of care without demonstrating cost effectiveness. Furthermore, many SNF directors might suggest that hospitals and hospitalists working with them to address fundamental (but much more intransigent) problems in SNFs, such as high staff turnover, low concentration of highly skilled staff (RNs and MDs), regulatory burden, and hospitals using SNFs like stepdown units, could represent a generalizable and sustainable solution.
We realize that this argument is tricky for hospitalists because its underlying logic (care has become too complex, patients are too sick, and dedicated personnel are needed) also played a major role in establishing our existence. One possibility is that like hospitalists, NPs and a growing cadre of “SNFists” will become major drivers of quality improvement, education, and leadership locally at these facilities, thereby leading to sustainable change.8 Similarly, current conditions may drive recognition that a specific set of skills is required to function effectively in the SNF environment,9 just as we believe hospitalists need unique skills to excel in today’s hospital environment.
Studies such as that of Rosen et al. are valuable for JHM because they prompt us to recognize that we as hospitalists have much to share and learn from nursing homes and the dedicated practitioners who work there. In fact, we argue that few places in the healthcare system are more in need of innovation than hospital–nursing home relationships, and hospitalists do not just have a vested clinical interest; in many ways, we see a mirror of our own development as a “specialty.” We encourage hospitals and hospitalists to take up this challenge on behalf of some of the most vulnerable patients in our system during critical times in their care trajectory. As the Commission for Long-Term Care (www.ltccommission.org) wrote in its final report to Congress: “The need is great. The time to act is now.”
Disclosures
Dr. Burke is supported by a VA Health Services Research and Development (HSR&D) Career Development Award. All opinions are those of the authors and do not necessarily represent those of the Department of Veterans Affairs. Dr. Greysen has nothing to disclose.
The landscape of postacute care in skilled nursing facilities (SNFs) in the United States is evolving. As the population ages, a growing number of elderly persons are being discharged to SNFs at an enormous cost and with clear evidence of disappointing outcomes. The reaction to these trends includes payment reforms that “bundle” hospital and postacute care, act as incentives to discourage SNFs, or penalize SNFs for undesired patient outcomes. Hospitalists are expected to increasingly feel the effect of these reforms.1
Thus, hospitals are demonstrating renewed interest in reducing readmissions from SNFs. In this issue of Journal of Hospital Medicine, Rosen and colleagues present the results of the Enhanced Care Program (ECP), a multicomponent intervention consisting of 9 nurse practitioners (NPs), a pharmacist, a pharmacy technician, a nurse educator, a program administrator, and a medical director.2 These providers are deployed to 8 SNFs around a large teaching hospital, providing direct clinical care as well as 24/7 call availability for enrolled patients, robust medication reconciliation, and monthly education for SNF nursing staff. A unique aspect of this model was that individual attending physicians in the associated SNFs could decide whether to enroll their patients in the model; patients not enrolled represented a contemporaneous control cohort. The authors found a nearly 30% reduction in the odds of 30-day readmission (OR 0.71 [0.60–0.85] after adjustment), which was robust to multiple sensitivity analyses, including a propensity-matched cohort comparison. The authors should be commended for working to mitigate these potential confounders, thereby strengthening their conclusions. Such a large reduction in readmissions reflects their high underlying prevalence (23% in the nonintervention cohort).
This report closely follows the evaluation of a similar program at the Cleveland Clinic called Connected Care Model (CCM), in which 4 physicians and 5 NPs or physician assistants provided care, including 24/7 call availability, in 7 associated SNFs.3 In a retrospective pre-post analysis comparing the 30-day readmission rates of these SNFs with those of others in the network, similar reductions in readmissions were observed. ECP and CCM represent important extensions of a much larger body of evidence, from the Evercare model4 to the Initiative to Reduce Avoidable Hospitalizations demonstration project, which suggests that adding NPs to nursing homes reduces hospitalizations.5
However, several factors have to be considered before disseminating ECP or CCM. First, other promising “proof of concept” quality improvement studies were not efficacious when rigorously tested in nursing homes.6 Second, these programs are representative of large academic medical centers, which may establish different relationships with different SNFs compared with smaller or less well-resourced hospitals. As the Initiative to Reduce Hospitalizations demonstrated, even a fundamentally similar intervention can have extremely different results depending on the nursing homes involved,5 and the science behind establishing effective hospital–SNF partnerships is still in its infancy.7 Third, both studies have significant methodological limitations, including most importantly that they are conducted within SNFs selected to be part of their hospitals’ network.
These significant early efforts also present an opportunity to reconsider the underlying assumption of these models: that adding more supervisory clinicians to SNFs is the right approach to reduce hospitalizations. Although adding resources is an attractive “plug and play” solution for many problems in healthcare delivery, placing only 1 NP in each of the 15,583 certified nursing facilities in the United States would employ fully 10% of the entire NP workforce. Amid rising concerns about costs related to our aging population, these interventions face substantial headwinds toward becoming the standard of care without demonstrating cost effectiveness. Furthermore, many SNF directors might suggest that hospitals and hospitalists working with them to address fundamental (but much more intransigent) problems in SNFs, such as high staff turnover, low concentration of highly skilled staff (RNs and MDs), regulatory burden, and hospitals using SNFs like stepdown units, could represent a generalizable and sustainable solution.
We realize that this argument is tricky for hospitalists because its underlying logic (care has become too complex, patients are too sick, and dedicated personnel are needed) also played a major role in establishing our existence. One possibility is that like hospitalists, NPs and a growing cadre of “SNFists” will become major drivers of quality improvement, education, and leadership locally at these facilities, thereby leading to sustainable change.8 Similarly, current conditions may drive recognition that a specific set of skills is required to function effectively in the SNF environment,9 just as we believe hospitalists need unique skills to excel in today’s hospital environment.
Studies such as that of Rosen et al. are valuable for JHM because they prompt us to recognize that we as hospitalists have much to share and learn from nursing homes and the dedicated practitioners who work there. In fact, we argue that few places in the healthcare system are more in need of innovation than hospital–nursing home relationships, and hospitalists do not just have a vested clinical interest; in many ways, we see a mirror of our own development as a “specialty.” We encourage hospitals and hospitalists to take up this challenge on behalf of some of the most vulnerable patients in our system during critical times in their care trajectory. As the Commission for Long-Term Care (www.ltccommission.org) wrote in its final report to Congress: “The need is great. The time to act is now.”
Disclosures
Dr. Burke is supported by a VA Health Services Research and Development (HSR&D) Career Development Award. All opinions are those of the authors and do not necessarily represent those of the Department of Veterans Affairs. Dr. Greysen has nothing to disclose.
1. Burke RE, Cumbler E, Coleman EA, Levy C. Post-acute care reform: Implications and opportunities for hospitalists. J Hosp Med. 2017;12(1):46-51. 10.3810/hp.2012.02.958. PubMed
2. Rosen BT, Halbert RJ, Hart K, Diniz MA, Isonaka S, Black JT. The enhanced care program: Impact of a care transitions program on 30-day hospital readmissions for patients discharged from an acute care facility to skilled nursing facilities. J Hosp Med. 2018;13(4):229-235.
3. Rothberg MB. Impact of a connected care model on 30-day readmission rates from skilled nursing facilities. J Hosp Med. 2017;12(4):238-244. 10.12788/jhm.2710. PubMed
4. Kane RL, Keckhafer G, Flood S, Bershadsky B, Siadaty MS. The effect of Evercare on hospital use. J Am Geriatr Soc. 2003;51(10):1427-1434. 10.1046/j.1532-5415.2003.51461.x. PubMed
5. Ingber MJ, Feng Z, Khatutsky G, et al. Initiative to reduce avoidable hospitalizations among nursing facility residents shows promising results. Health Aff Proj Hope. 2017;36(3):441-450. 10.1377/hlthaff.2016.1310. PubMed
6. Kane RL, Huckfeldt P, Tappen R, et al. Effects of an intervention to reduce hospitalizations from nursing homes: A randomized implementation trial of the INTERACT Program. JAMA Intern Med. 2017;177(9):1257-1264. 10.1001/jamainternmed.2017.2657. PubMed
7. Lage DE, Rusinak D, Carr D, Grabowski DC, Ackerly DC. Creating a network of high-quality skilled nursing facilities: preliminary data on the postacute care quality improvement experiences of an accountable care organization. J Am Geriatr Soc. 2015;63(4):804-808. 10.1111/jgs.13351. PubMed
8. Ryskina KL, Polsky D, Werner RM. Physicians and advanced practitioners specializing in nursing home care. JAMA. 2017;318(20):2040-2042. 10.1001/jama.2017.13378. PubMed
9. Gillespie SM, Levy CR, Katz PR. What exactly is an “SNF-ist?” JAMA Intern Med. 2018;178(1):153-154. 10.1001/jamainternmed.2017.7212. PubMed
1. Burke RE, Cumbler E, Coleman EA, Levy C. Post-acute care reform: Implications and opportunities for hospitalists. J Hosp Med. 2017;12(1):46-51. 10.3810/hp.2012.02.958. PubMed
2. Rosen BT, Halbert RJ, Hart K, Diniz MA, Isonaka S, Black JT. The enhanced care program: Impact of a care transitions program on 30-day hospital readmissions for patients discharged from an acute care facility to skilled nursing facilities. J Hosp Med. 2018;13(4):229-235.
3. Rothberg MB. Impact of a connected care model on 30-day readmission rates from skilled nursing facilities. J Hosp Med. 2017;12(4):238-244. 10.12788/jhm.2710. PubMed
4. Kane RL, Keckhafer G, Flood S, Bershadsky B, Siadaty MS. The effect of Evercare on hospital use. J Am Geriatr Soc. 2003;51(10):1427-1434. 10.1046/j.1532-5415.2003.51461.x. PubMed
5. Ingber MJ, Feng Z, Khatutsky G, et al. Initiative to reduce avoidable hospitalizations among nursing facility residents shows promising results. Health Aff Proj Hope. 2017;36(3):441-450. 10.1377/hlthaff.2016.1310. PubMed
6. Kane RL, Huckfeldt P, Tappen R, et al. Effects of an intervention to reduce hospitalizations from nursing homes: A randomized implementation trial of the INTERACT Program. JAMA Intern Med. 2017;177(9):1257-1264. 10.1001/jamainternmed.2017.2657. PubMed
7. Lage DE, Rusinak D, Carr D, Grabowski DC, Ackerly DC. Creating a network of high-quality skilled nursing facilities: preliminary data on the postacute care quality improvement experiences of an accountable care organization. J Am Geriatr Soc. 2015;63(4):804-808. 10.1111/jgs.13351. PubMed
8. Ryskina KL, Polsky D, Werner RM. Physicians and advanced practitioners specializing in nursing home care. JAMA. 2017;318(20):2040-2042. 10.1001/jama.2017.13378. PubMed
9. Gillespie SM, Levy CR, Katz PR. What exactly is an “SNF-ist?” JAMA Intern Med. 2018;178(1):153-154. 10.1001/jamainternmed.2017.7212. PubMed
© 2018 Society of Hospital Medicine
Hemodynamically, the kidney is at the heart of cardiorenal syndrome
In heart failure, the heart and the kidneys share a rocky relationship. Cardiac dysfunction can heighten renal dysfunction and vice versa—appropriately dubbed “cardiorenal syndrome.”
Although classically defined by a reduction in the glomerular filtration rate (GFR),1 cardiorenal syndrome also encompasses complex neurohormonal, pharmacologic, and metabolic interactions affecting or affected by both glomerular and tubular function. Unfortunately, all of these maladaptive processes occur in heart failure and perpetuate a vicious circle of continued dual-organ dysfunction.
The central insult here is hemodynamic disarray from acute or chronic cardiac dysfunction, which can directly influence glomerular function. However, to understand the hemodynamic ramifications for glomerular function, we focus on the determinants of glomerular filtration.
DETERMINANTS OF GFR
The GFR is the rate of fluid flow between the glomerular capillaries and the Bowman capsule and is classically represented by the following equations2:
GFR = Kf × (PG – PB – πG + πB)
Kf = N × Lp × S
Kf is the filtration constant, N the number of functional nephrons, Lp the hydraulic conductivity of the glomerular capillary, S the filtration area, PG the hydrostatic pressure in the glomerular capillaries, PB the hydrostatic pressure in the Bowman capsule, and πG and πB the colloid osmotic pressures within the glomerular capillaries and Bowman space, respectively.
Based on this relationship, the GFR is reduced when PG is reduced in the setting of hypovolemia, hypotension, or renin-angiotensin system antagonist use or when PB is increased in the setting of elevated central venous pressure or elevated abdominal pressure—all common in heart failure. With this understanding, one would assume that strategies to increase PG (improve perfusion) and reduce PB (reduce congestion) might ameliorate ongoing renal dysfunction and improve the GFR in heart failure.
In this issue, Thind et al3 highlight the impact of hemodynamic derangements in heart failure with acute cardiorenal syndrome and provide an overview of its treatment. They review the complex relationship between progressive cardiac failure translating into accelerated neurohormonal responses (increases in sympathetic nervous system and renin-angiotensin-aldosterone system activation) and the impact of increased central venous pressure on progressive renal dysfunction. They also provide an overview of efforts to mitigate cardiorenal syndrome, after careful appraisal of volume status, through diuretic-mediated decongestion with aggressive use of loop diuretics (either in isolation or in the form of sequential nephron blockade with a thiazide or acetazolamide), and they highlight the lingering uncertainty regarding inotrope use.
VENOUS CONGESTION VS DECREASED CARDIAC OUTPUT
Returning to the GFR equation, it is clear that an imbalance in PG and PB can worsen glomerular function. Because cardiac dysfunction can lead to both venous congestion and decreased cardiac output, this leads to the question, “Of these, which is the more important driver of this imbalance and its effects on renal function?”
A compelling argument can be made for each side. On one hand, experiments over a half-century old in human models of venous congestion highlighted the profound impact of elevated venous pressure, which decreases electrolyte excretion (sodium included) and diminishes urine flow.4,5 This has been replicated in more-contemporary decompensated heart failure cohorts in which worsening renal function was more closely associated with elevated central venous pressure rather than cardiac output.6,7 On the other hand, early landmark experiments and more recent cohorts with heart failure have also shown that reductions in effective arterial blood volume, renal blood flow, and cardiac output are also associated with reductions in GFR.5,8,9
How then shall we reconcile whether cardiorenal syndrome is a “backward failure” (from central venous pressure) or a “forward failure” (from decreased perfusion) phenomenon?
The answer is complicated and is likely “both,” with the major component being increased central venous pressure. To understand this construct, we must first exclude frank cardiogenic shock—when the hydraulic function of the heart fails to provide enough flow, leading to a catastrophic drop in mean arterial pressure that supersedes the kidney’s ability to autoregulate renal blood flow.10,11
In patients with chronic heart failure and congestion who are not in shock, historical observations suggest that both intra-abdominal pressure (which increases renal venous pressure) and central venous pressure lead to reduced renal blood flow and increased renal vasomotor resistance (increase in afferent, intrarenal, and efferent vascular tone).12–14 More recent observations from epidemiologic studies have largely replicated these findings. Central venous pressure remains essential to impacting renal function in heart failure,6,15 and the impact of cardiac output on renal function remains uncertain.16
The relationship of intracardiac hemodynamics may also play a role in modifying renal function. Several reports recently described the relationship between both right- and left-sided filling pressures as being associated with worse renal function in heart failure.17–19 Patients with a disproportionately higher right atrial pressure to pulmonary capillary wedge pressure have higher serum creatinine during and after decongestive therapies. Therefore, the concept of “right-sided heart failure” expands beyond the simple representation of “backward congestion” at the level of venous return. In fact, a higher ratio of right atrial pressure to pulmonary capillary wedge pressure may point to an inability of the venous and pulmonary circulations to provide adequate left ventricular preload. Therefore, a relatively underfilled left ventricle in the face of biventricular dysfunction may result in worsening renal function.
TREATMENT IS CHALLENGING
The treatment of cardiorenal syndrome is challenging. It is often accompanied by heightened azotemia, diuretic resistance, electrolyte abnormalities, and a spectrum of hemodynamic disarray. As Thind et al point out, there is, unfortunately, no firmly established treatment. While “sequential nephron blockade” (pharmacologically blocking multiple sites on the nephron simultaneously) is theoretically promising, there are no rigorously studied therapeutic strategies with proven efficacy.
On the other hand, mechanical removal of isotonic fluid with ultrafiltration showed early promise in decompensated heart failure, but enthusiasm diminished with results from the Cardiorenal Rescue Study in Acute Decompensated Heart Failure (CARRESS-HF) trial.20 Ultrafiltration was roughly equivalent to aggressive pharmacologic therapy for fluid loss, was associated with higher serum creatinine levels, and was more challenging to administer.
Equally uncertain is the benefit of inotropic or vasoactive therapy, which directly alters cardiac hemodynamics. Low-dose dopamine or low-dose nesiritide is of no benefit toward enhancement of decongestion or renal protection when added to standard diuretic therapy.21 Furthermore, routine use of inotropes is fraught with more arrhythmias and hypotension and is associated with dismal long-term outcomes.22,23
Alternative therapies that act directly on renal physiology—eg, rolofylline, a selective adenosine A1 receptor antagonist that may enhance renal blood flow, augment natriuresis, and break diuretic resistance—have been similarly disappointing.24
With so much uncertainty, more investigation into novel treatments for cardiorenal syndrome is clearly warranted.
However, because venous congestion is the hemodynamic hallmark of acute cardiorenal syndrome (increasing PB), reducing central venous pressure remains the cornerstone treatment for cardiorenal syndrome. Additionally, efforts to preserve renal perfusion and avoid hypotension are prudent to maintain glomerular capillary hydrostatic pressure (PG).
In light of these considerations, there is no “one size fits all” for the treatment of cardiorenal syndrome. Treatment should be based on thoughtful individualized strategies tailored to the underlying cardiorenal pathophysiology, and with the understanding that the kidney is at the heart of the matter.
- House AA, Anand I, Bellomo R, et al; Acute Dialysis Quality Initiative Consensus Group. Definition and classification of cardio-renal syndromes: workgroup statements from the 7th ADQI Consensus Conference. Nephrol Dial Transplant 2010; 25:1416–1420.
- Tucker BJ, Blantz RC. An analysis of the determinants of nephron filtration rate. Am J Physiol 1977; 232:F477–F483.
- Thind GS, Loehrke M, Wilt JL. Acute cardiorenal syndrome: mechanisms and clinical implications. Cleve Clin J Med 2018; 85:231–239.
- Wilkins RW, Tinsley CM, Culbertson JW, et al. The effects of venous congestion of the limbs upon renal clearances and the excretion of water and salt. I. Studies in normal subjects and in hypertensive patients before and after splanchnicectomy. J Clin Invest 1953; 32:1101–1116.
- Judson WE, Hatcher JD, Hollander W, Halperin MH, Wilkins RW. The effects of venous congestion of the limbs and phlebotomy upon renal clearances and the excretion of water and salt. II. Studies in patients with congestive failure. J Clin Invest 1955; 34:1591–1599.
- Mullens W, Abrahams Z, Francis GS, et al. Importance of venous congestion for worsening of renal function in advanced decompensated heart failure. J Am Coll Cardiol 2009; 53:589–596.
- Damman K, van Deursen VM, Navis G, Voors AA, van Veldhuisen DJ, Hillege HL. Increased central venous pressure is associated with impaired renal function and mortality in a broad spectrum of patients with cardiovascular disease. J Am Coll Cardiol 2009; 53:582–588.
- Ljungman S, Laragh JH, Cody RJ. Role of the kidney in congestive heart failure. Relationship of cardiac index to kidney function. Drugs 1990; 39(suppl 4):10–21; discussion 22–24.
- Damman K, Navis G, Smilde TD, et al. Decreased cardiac output, venous congestion and the association with renal impairment in patients with cardiac dysfunction. Eur J Heart Fail 2007; 9:872–878.
- Fincke R, Hochman JS, Lowe AM, et al. Cardiac power is the strongest hemodynamic correlate of mortality in cardiogenic shock: a report from the SHOCK trial registry. J Am Coll Cardiol 2004; 44:340–348.
- Adams PL, Adams FF, Bell PD, Navar LG. Impaired renal blood flow autoregulation in ischemic acute renal failure. Kidney Int 1980; 18:68–76.
- Maxwell MH, Breed ES, Schwartz IL. Renal venous pressure in chronic congestive heart failure. J Clin Invest 1950; 29:342–348.
- Blake WD, Wégria R, Keating RP, Ward HP. Effect of increased renal venous pressure on renal function. Am J Physiol 1949; 157:1–13.
- Bradley SE, Bradley GP. The effect of increased intra-abdominal pressure on renal function in man. J Clin Invest 1947; 26:1010–1022.
- Mullens W, Abrahams Z, Skouri HN, et al. Elevated intra-abdominal pressure in acute decompensated heart failure: a potential contributor to worsening renal function? J Am Coll Cardiol 2008; 51:300–306.
- Hanberg JS, Sury K, Wilson FP, et al. Reduced cardiac index is not the dominant driver of renal dysfunction in heart failure. J Am Coll Cardiol 2016; 67:2199–2208.
- Drazner MH, Brown RN, Kaiser PA, et al. Relationship of right- and left-sided filling pressures in patients with advanced heart failure: a 14-year multi-institutional analysis. J Heart Lung Transplant 2012; 31:67–72.
- Drazner MH, Velez-Martinez M, Ayers CR, et al. Relationship of right- to left-sided ventricular filling pressures in advanced heart failure: insights from the ESCAPE trial. Circ Heart Fail 2013; 6:264–270.
- Grodin JL, Drazner MH, Dupont M, et al. A disproportionate elevation in right ventricular filling pressure, in relation to left ventricular filling pressure, is associated with renal impairment and increased mortality in advanced decompensated heart failure. Am Heart J 2015; 169:806–812.
- Bart BA, Goldsmith SR, Lee KL, et al, for the Heart Failure Clinical Research Network. Ultrafiltration in decompensated heart failure with cardiorenal syndrome. N Engl J Med 2012; 367:2296–2304.
- Chen HH, Anstrom KJ, Givertz MM, et al; NHLBI Heart Failure Clinical Research Network. Low-dose dopamine or low-dose nesiritide in acute heart failure with renal dysfunction: the ROSE acute heart failure randomized trial. JAMA 2013; 310:2533–2543.
- Gorodeski EZ, Chu EC, Reese JR, Shishehbor MH, Hsich E, Starling RC. Prognosis on chronic dobutamine or milrinone infusions for stage D heart failure. Circ Heart Fail 2009; 2:320–324.
- Cuffe MS, Califf RM, Adams KF Jr, et al, for the Outcomes of a Prospective Trial of Intravenous Milrinone for Exacerbations of Chronic Heart Failure (OPTIME-CHF) Investigators. Short-term intravenous milrinone for acute exacerbation of chronic heart failure: a randomized controlled trial. JAMA 2002; 287:1541–1547.
- Massie BM, O’Connor CM, Metra M, et al, for the PROTECT Investigators and Committees. Rolofylline, an adenosine A1-receptor antagonist, in acute heart failure. N Engl J Med 2010; 363:1419–1428.
In heart failure, the heart and the kidneys share a rocky relationship. Cardiac dysfunction can heighten renal dysfunction and vice versa—appropriately dubbed “cardiorenal syndrome.”
Although classically defined by a reduction in the glomerular filtration rate (GFR),1 cardiorenal syndrome also encompasses complex neurohormonal, pharmacologic, and metabolic interactions affecting or affected by both glomerular and tubular function. Unfortunately, all of these maladaptive processes occur in heart failure and perpetuate a vicious circle of continued dual-organ dysfunction.
The central insult here is hemodynamic disarray from acute or chronic cardiac dysfunction, which can directly influence glomerular function. However, to understand the hemodynamic ramifications for glomerular function, we focus on the determinants of glomerular filtration.
DETERMINANTS OF GFR
The GFR is the rate of fluid flow between the glomerular capillaries and the Bowman capsule and is classically represented by the following equations2:
GFR = Kf × (PG – PB – πG + πB)
Kf = N × Lp × S
Kf is the filtration constant, N the number of functional nephrons, Lp the hydraulic conductivity of the glomerular capillary, S the filtration area, PG the hydrostatic pressure in the glomerular capillaries, PB the hydrostatic pressure in the Bowman capsule, and πG and πB the colloid osmotic pressures within the glomerular capillaries and Bowman space, respectively.
Based on this relationship, the GFR is reduced when PG is reduced in the setting of hypovolemia, hypotension, or renin-angiotensin system antagonist use or when PB is increased in the setting of elevated central venous pressure or elevated abdominal pressure—all common in heart failure. With this understanding, one would assume that strategies to increase PG (improve perfusion) and reduce PB (reduce congestion) might ameliorate ongoing renal dysfunction and improve the GFR in heart failure.
In this issue, Thind et al3 highlight the impact of hemodynamic derangements in heart failure with acute cardiorenal syndrome and provide an overview of its treatment. They review the complex relationship between progressive cardiac failure translating into accelerated neurohormonal responses (increases in sympathetic nervous system and renin-angiotensin-aldosterone system activation) and the impact of increased central venous pressure on progressive renal dysfunction. They also provide an overview of efforts to mitigate cardiorenal syndrome, after careful appraisal of volume status, through diuretic-mediated decongestion with aggressive use of loop diuretics (either in isolation or in the form of sequential nephron blockade with a thiazide or acetazolamide), and they highlight the lingering uncertainty regarding inotrope use.
VENOUS CONGESTION VS DECREASED CARDIAC OUTPUT
Returning to the GFR equation, it is clear that an imbalance in PG and PB can worsen glomerular function. Because cardiac dysfunction can lead to both venous congestion and decreased cardiac output, this leads to the question, “Of these, which is the more important driver of this imbalance and its effects on renal function?”
A compelling argument can be made for each side. On one hand, experiments over a half-century old in human models of venous congestion highlighted the profound impact of elevated venous pressure, which decreases electrolyte excretion (sodium included) and diminishes urine flow.4,5 This has been replicated in more-contemporary decompensated heart failure cohorts in which worsening renal function was more closely associated with elevated central venous pressure rather than cardiac output.6,7 On the other hand, early landmark experiments and more recent cohorts with heart failure have also shown that reductions in effective arterial blood volume, renal blood flow, and cardiac output are also associated with reductions in GFR.5,8,9
How then shall we reconcile whether cardiorenal syndrome is a “backward failure” (from central venous pressure) or a “forward failure” (from decreased perfusion) phenomenon?
The answer is complicated and is likely “both,” with the major component being increased central venous pressure. To understand this construct, we must first exclude frank cardiogenic shock—when the hydraulic function of the heart fails to provide enough flow, leading to a catastrophic drop in mean arterial pressure that supersedes the kidney’s ability to autoregulate renal blood flow.10,11
In patients with chronic heart failure and congestion who are not in shock, historical observations suggest that both intra-abdominal pressure (which increases renal venous pressure) and central venous pressure lead to reduced renal blood flow and increased renal vasomotor resistance (increase in afferent, intrarenal, and efferent vascular tone).12–14 More recent observations from epidemiologic studies have largely replicated these findings. Central venous pressure remains essential to impacting renal function in heart failure,6,15 and the impact of cardiac output on renal function remains uncertain.16
The relationship of intracardiac hemodynamics may also play a role in modifying renal function. Several reports recently described the relationship between both right- and left-sided filling pressures as being associated with worse renal function in heart failure.17–19 Patients with a disproportionately higher right atrial pressure to pulmonary capillary wedge pressure have higher serum creatinine during and after decongestive therapies. Therefore, the concept of “right-sided heart failure” expands beyond the simple representation of “backward congestion” at the level of venous return. In fact, a higher ratio of right atrial pressure to pulmonary capillary wedge pressure may point to an inability of the venous and pulmonary circulations to provide adequate left ventricular preload. Therefore, a relatively underfilled left ventricle in the face of biventricular dysfunction may result in worsening renal function.
TREATMENT IS CHALLENGING
The treatment of cardiorenal syndrome is challenging. It is often accompanied by heightened azotemia, diuretic resistance, electrolyte abnormalities, and a spectrum of hemodynamic disarray. As Thind et al point out, there is, unfortunately, no firmly established treatment. While “sequential nephron blockade” (pharmacologically blocking multiple sites on the nephron simultaneously) is theoretically promising, there are no rigorously studied therapeutic strategies with proven efficacy.
On the other hand, mechanical removal of isotonic fluid with ultrafiltration showed early promise in decompensated heart failure, but enthusiasm diminished with results from the Cardiorenal Rescue Study in Acute Decompensated Heart Failure (CARRESS-HF) trial.20 Ultrafiltration was roughly equivalent to aggressive pharmacologic therapy for fluid loss, was associated with higher serum creatinine levels, and was more challenging to administer.
Equally uncertain is the benefit of inotropic or vasoactive therapy, which directly alters cardiac hemodynamics. Low-dose dopamine or low-dose nesiritide is of no benefit toward enhancement of decongestion or renal protection when added to standard diuretic therapy.21 Furthermore, routine use of inotropes is fraught with more arrhythmias and hypotension and is associated with dismal long-term outcomes.22,23
Alternative therapies that act directly on renal physiology—eg, rolofylline, a selective adenosine A1 receptor antagonist that may enhance renal blood flow, augment natriuresis, and break diuretic resistance—have been similarly disappointing.24
With so much uncertainty, more investigation into novel treatments for cardiorenal syndrome is clearly warranted.
However, because venous congestion is the hemodynamic hallmark of acute cardiorenal syndrome (increasing PB), reducing central venous pressure remains the cornerstone treatment for cardiorenal syndrome. Additionally, efforts to preserve renal perfusion and avoid hypotension are prudent to maintain glomerular capillary hydrostatic pressure (PG).
In light of these considerations, there is no “one size fits all” for the treatment of cardiorenal syndrome. Treatment should be based on thoughtful individualized strategies tailored to the underlying cardiorenal pathophysiology, and with the understanding that the kidney is at the heart of the matter.
In heart failure, the heart and the kidneys share a rocky relationship. Cardiac dysfunction can heighten renal dysfunction and vice versa—appropriately dubbed “cardiorenal syndrome.”
Although classically defined by a reduction in the glomerular filtration rate (GFR),1 cardiorenal syndrome also encompasses complex neurohormonal, pharmacologic, and metabolic interactions affecting or affected by both glomerular and tubular function. Unfortunately, all of these maladaptive processes occur in heart failure and perpetuate a vicious circle of continued dual-organ dysfunction.
The central insult here is hemodynamic disarray from acute or chronic cardiac dysfunction, which can directly influence glomerular function. However, to understand the hemodynamic ramifications for glomerular function, we focus on the determinants of glomerular filtration.
DETERMINANTS OF GFR
The GFR is the rate of fluid flow between the glomerular capillaries and the Bowman capsule and is classically represented by the following equations2:
GFR = Kf × (PG – PB – πG + πB)
Kf = N × Lp × S
Kf is the filtration constant, N the number of functional nephrons, Lp the hydraulic conductivity of the glomerular capillary, S the filtration area, PG the hydrostatic pressure in the glomerular capillaries, PB the hydrostatic pressure in the Bowman capsule, and πG and πB the colloid osmotic pressures within the glomerular capillaries and Bowman space, respectively.
Based on this relationship, the GFR is reduced when PG is reduced in the setting of hypovolemia, hypotension, or renin-angiotensin system antagonist use or when PB is increased in the setting of elevated central venous pressure or elevated abdominal pressure—all common in heart failure. With this understanding, one would assume that strategies to increase PG (improve perfusion) and reduce PB (reduce congestion) might ameliorate ongoing renal dysfunction and improve the GFR in heart failure.
In this issue, Thind et al3 highlight the impact of hemodynamic derangements in heart failure with acute cardiorenal syndrome and provide an overview of its treatment. They review the complex relationship between progressive cardiac failure translating into accelerated neurohormonal responses (increases in sympathetic nervous system and renin-angiotensin-aldosterone system activation) and the impact of increased central venous pressure on progressive renal dysfunction. They also provide an overview of efforts to mitigate cardiorenal syndrome, after careful appraisal of volume status, through diuretic-mediated decongestion with aggressive use of loop diuretics (either in isolation or in the form of sequential nephron blockade with a thiazide or acetazolamide), and they highlight the lingering uncertainty regarding inotrope use.
VENOUS CONGESTION VS DECREASED CARDIAC OUTPUT
Returning to the GFR equation, it is clear that an imbalance in PG and PB can worsen glomerular function. Because cardiac dysfunction can lead to both venous congestion and decreased cardiac output, this leads to the question, “Of these, which is the more important driver of this imbalance and its effects on renal function?”
A compelling argument can be made for each side. On one hand, experiments over a half-century old in human models of venous congestion highlighted the profound impact of elevated venous pressure, which decreases electrolyte excretion (sodium included) and diminishes urine flow.4,5 This has been replicated in more-contemporary decompensated heart failure cohorts in which worsening renal function was more closely associated with elevated central venous pressure rather than cardiac output.6,7 On the other hand, early landmark experiments and more recent cohorts with heart failure have also shown that reductions in effective arterial blood volume, renal blood flow, and cardiac output are also associated with reductions in GFR.5,8,9
How then shall we reconcile whether cardiorenal syndrome is a “backward failure” (from central venous pressure) or a “forward failure” (from decreased perfusion) phenomenon?
The answer is complicated and is likely “both,” with the major component being increased central venous pressure. To understand this construct, we must first exclude frank cardiogenic shock—when the hydraulic function of the heart fails to provide enough flow, leading to a catastrophic drop in mean arterial pressure that supersedes the kidney’s ability to autoregulate renal blood flow.10,11
In patients with chronic heart failure and congestion who are not in shock, historical observations suggest that both intra-abdominal pressure (which increases renal venous pressure) and central venous pressure lead to reduced renal blood flow and increased renal vasomotor resistance (increase in afferent, intrarenal, and efferent vascular tone).12–14 More recent observations from epidemiologic studies have largely replicated these findings. Central venous pressure remains essential to impacting renal function in heart failure,6,15 and the impact of cardiac output on renal function remains uncertain.16
The relationship of intracardiac hemodynamics may also play a role in modifying renal function. Several reports recently described the relationship between both right- and left-sided filling pressures as being associated with worse renal function in heart failure.17–19 Patients with a disproportionately higher right atrial pressure to pulmonary capillary wedge pressure have higher serum creatinine during and after decongestive therapies. Therefore, the concept of “right-sided heart failure” expands beyond the simple representation of “backward congestion” at the level of venous return. In fact, a higher ratio of right atrial pressure to pulmonary capillary wedge pressure may point to an inability of the venous and pulmonary circulations to provide adequate left ventricular preload. Therefore, a relatively underfilled left ventricle in the face of biventricular dysfunction may result in worsening renal function.
TREATMENT IS CHALLENGING
The treatment of cardiorenal syndrome is challenging. It is often accompanied by heightened azotemia, diuretic resistance, electrolyte abnormalities, and a spectrum of hemodynamic disarray. As Thind et al point out, there is, unfortunately, no firmly established treatment. While “sequential nephron blockade” (pharmacologically blocking multiple sites on the nephron simultaneously) is theoretically promising, there are no rigorously studied therapeutic strategies with proven efficacy.
On the other hand, mechanical removal of isotonic fluid with ultrafiltration showed early promise in decompensated heart failure, but enthusiasm diminished with results from the Cardiorenal Rescue Study in Acute Decompensated Heart Failure (CARRESS-HF) trial.20 Ultrafiltration was roughly equivalent to aggressive pharmacologic therapy for fluid loss, was associated with higher serum creatinine levels, and was more challenging to administer.
Equally uncertain is the benefit of inotropic or vasoactive therapy, which directly alters cardiac hemodynamics. Low-dose dopamine or low-dose nesiritide is of no benefit toward enhancement of decongestion or renal protection when added to standard diuretic therapy.21 Furthermore, routine use of inotropes is fraught with more arrhythmias and hypotension and is associated with dismal long-term outcomes.22,23
Alternative therapies that act directly on renal physiology—eg, rolofylline, a selective adenosine A1 receptor antagonist that may enhance renal blood flow, augment natriuresis, and break diuretic resistance—have been similarly disappointing.24
With so much uncertainty, more investigation into novel treatments for cardiorenal syndrome is clearly warranted.
However, because venous congestion is the hemodynamic hallmark of acute cardiorenal syndrome (increasing PB), reducing central venous pressure remains the cornerstone treatment for cardiorenal syndrome. Additionally, efforts to preserve renal perfusion and avoid hypotension are prudent to maintain glomerular capillary hydrostatic pressure (PG).
In light of these considerations, there is no “one size fits all” for the treatment of cardiorenal syndrome. Treatment should be based on thoughtful individualized strategies tailored to the underlying cardiorenal pathophysiology, and with the understanding that the kidney is at the heart of the matter.
- House AA, Anand I, Bellomo R, et al; Acute Dialysis Quality Initiative Consensus Group. Definition and classification of cardio-renal syndromes: workgroup statements from the 7th ADQI Consensus Conference. Nephrol Dial Transplant 2010; 25:1416–1420.
- Tucker BJ, Blantz RC. An analysis of the determinants of nephron filtration rate. Am J Physiol 1977; 232:F477–F483.
- Thind GS, Loehrke M, Wilt JL. Acute cardiorenal syndrome: mechanisms and clinical implications. Cleve Clin J Med 2018; 85:231–239.
- Wilkins RW, Tinsley CM, Culbertson JW, et al. The effects of venous congestion of the limbs upon renal clearances and the excretion of water and salt. I. Studies in normal subjects and in hypertensive patients before and after splanchnicectomy. J Clin Invest 1953; 32:1101–1116.
- Judson WE, Hatcher JD, Hollander W, Halperin MH, Wilkins RW. The effects of venous congestion of the limbs and phlebotomy upon renal clearances and the excretion of water and salt. II. Studies in patients with congestive failure. J Clin Invest 1955; 34:1591–1599.
- Mullens W, Abrahams Z, Francis GS, et al. Importance of venous congestion for worsening of renal function in advanced decompensated heart failure. J Am Coll Cardiol 2009; 53:589–596.
- Damman K, van Deursen VM, Navis G, Voors AA, van Veldhuisen DJ, Hillege HL. Increased central venous pressure is associated with impaired renal function and mortality in a broad spectrum of patients with cardiovascular disease. J Am Coll Cardiol 2009; 53:582–588.
- Ljungman S, Laragh JH, Cody RJ. Role of the kidney in congestive heart failure. Relationship of cardiac index to kidney function. Drugs 1990; 39(suppl 4):10–21; discussion 22–24.
- Damman K, Navis G, Smilde TD, et al. Decreased cardiac output, venous congestion and the association with renal impairment in patients with cardiac dysfunction. Eur J Heart Fail 2007; 9:872–878.
- Fincke R, Hochman JS, Lowe AM, et al. Cardiac power is the strongest hemodynamic correlate of mortality in cardiogenic shock: a report from the SHOCK trial registry. J Am Coll Cardiol 2004; 44:340–348.
- Adams PL, Adams FF, Bell PD, Navar LG. Impaired renal blood flow autoregulation in ischemic acute renal failure. Kidney Int 1980; 18:68–76.
- Maxwell MH, Breed ES, Schwartz IL. Renal venous pressure in chronic congestive heart failure. J Clin Invest 1950; 29:342–348.
- Blake WD, Wégria R, Keating RP, Ward HP. Effect of increased renal venous pressure on renal function. Am J Physiol 1949; 157:1–13.
- Bradley SE, Bradley GP. The effect of increased intra-abdominal pressure on renal function in man. J Clin Invest 1947; 26:1010–1022.
- Mullens W, Abrahams Z, Skouri HN, et al. Elevated intra-abdominal pressure in acute decompensated heart failure: a potential contributor to worsening renal function? J Am Coll Cardiol 2008; 51:300–306.
- Hanberg JS, Sury K, Wilson FP, et al. Reduced cardiac index is not the dominant driver of renal dysfunction in heart failure. J Am Coll Cardiol 2016; 67:2199–2208.
- Drazner MH, Brown RN, Kaiser PA, et al. Relationship of right- and left-sided filling pressures in patients with advanced heart failure: a 14-year multi-institutional analysis. J Heart Lung Transplant 2012; 31:67–72.
- Drazner MH, Velez-Martinez M, Ayers CR, et al. Relationship of right- to left-sided ventricular filling pressures in advanced heart failure: insights from the ESCAPE trial. Circ Heart Fail 2013; 6:264–270.
- Grodin JL, Drazner MH, Dupont M, et al. A disproportionate elevation in right ventricular filling pressure, in relation to left ventricular filling pressure, is associated with renal impairment and increased mortality in advanced decompensated heart failure. Am Heart J 2015; 169:806–812.
- Bart BA, Goldsmith SR, Lee KL, et al, for the Heart Failure Clinical Research Network. Ultrafiltration in decompensated heart failure with cardiorenal syndrome. N Engl J Med 2012; 367:2296–2304.
- Chen HH, Anstrom KJ, Givertz MM, et al; NHLBI Heart Failure Clinical Research Network. Low-dose dopamine or low-dose nesiritide in acute heart failure with renal dysfunction: the ROSE acute heart failure randomized trial. JAMA 2013; 310:2533–2543.
- Gorodeski EZ, Chu EC, Reese JR, Shishehbor MH, Hsich E, Starling RC. Prognosis on chronic dobutamine or milrinone infusions for stage D heart failure. Circ Heart Fail 2009; 2:320–324.
- Cuffe MS, Califf RM, Adams KF Jr, et al, for the Outcomes of a Prospective Trial of Intravenous Milrinone for Exacerbations of Chronic Heart Failure (OPTIME-CHF) Investigators. Short-term intravenous milrinone for acute exacerbation of chronic heart failure: a randomized controlled trial. JAMA 2002; 287:1541–1547.
- Massie BM, O’Connor CM, Metra M, et al, for the PROTECT Investigators and Committees. Rolofylline, an adenosine A1-receptor antagonist, in acute heart failure. N Engl J Med 2010; 363:1419–1428.
- House AA, Anand I, Bellomo R, et al; Acute Dialysis Quality Initiative Consensus Group. Definition and classification of cardio-renal syndromes: workgroup statements from the 7th ADQI Consensus Conference. Nephrol Dial Transplant 2010; 25:1416–1420.
- Tucker BJ, Blantz RC. An analysis of the determinants of nephron filtration rate. Am J Physiol 1977; 232:F477–F483.
- Thind GS, Loehrke M, Wilt JL. Acute cardiorenal syndrome: mechanisms and clinical implications. Cleve Clin J Med 2018; 85:231–239.
- Wilkins RW, Tinsley CM, Culbertson JW, et al. The effects of venous congestion of the limbs upon renal clearances and the excretion of water and salt. I. Studies in normal subjects and in hypertensive patients before and after splanchnicectomy. J Clin Invest 1953; 32:1101–1116.
- Judson WE, Hatcher JD, Hollander W, Halperin MH, Wilkins RW. The effects of venous congestion of the limbs and phlebotomy upon renal clearances and the excretion of water and salt. II. Studies in patients with congestive failure. J Clin Invest 1955; 34:1591–1599.
- Mullens W, Abrahams Z, Francis GS, et al. Importance of venous congestion for worsening of renal function in advanced decompensated heart failure. J Am Coll Cardiol 2009; 53:589–596.
- Damman K, van Deursen VM, Navis G, Voors AA, van Veldhuisen DJ, Hillege HL. Increased central venous pressure is associated with impaired renal function and mortality in a broad spectrum of patients with cardiovascular disease. J Am Coll Cardiol 2009; 53:582–588.
- Ljungman S, Laragh JH, Cody RJ. Role of the kidney in congestive heart failure. Relationship of cardiac index to kidney function. Drugs 1990; 39(suppl 4):10–21; discussion 22–24.
- Damman K, Navis G, Smilde TD, et al. Decreased cardiac output, venous congestion and the association with renal impairment in patients with cardiac dysfunction. Eur J Heart Fail 2007; 9:872–878.
- Fincke R, Hochman JS, Lowe AM, et al. Cardiac power is the strongest hemodynamic correlate of mortality in cardiogenic shock: a report from the SHOCK trial registry. J Am Coll Cardiol 2004; 44:340–348.
- Adams PL, Adams FF, Bell PD, Navar LG. Impaired renal blood flow autoregulation in ischemic acute renal failure. Kidney Int 1980; 18:68–76.
- Maxwell MH, Breed ES, Schwartz IL. Renal venous pressure in chronic congestive heart failure. J Clin Invest 1950; 29:342–348.
- Blake WD, Wégria R, Keating RP, Ward HP. Effect of increased renal venous pressure on renal function. Am J Physiol 1949; 157:1–13.
- Bradley SE, Bradley GP. The effect of increased intra-abdominal pressure on renal function in man. J Clin Invest 1947; 26:1010–1022.
- Mullens W, Abrahams Z, Skouri HN, et al. Elevated intra-abdominal pressure in acute decompensated heart failure: a potential contributor to worsening renal function? J Am Coll Cardiol 2008; 51:300–306.
- Hanberg JS, Sury K, Wilson FP, et al. Reduced cardiac index is not the dominant driver of renal dysfunction in heart failure. J Am Coll Cardiol 2016; 67:2199–2208.
- Drazner MH, Brown RN, Kaiser PA, et al. Relationship of right- and left-sided filling pressures in patients with advanced heart failure: a 14-year multi-institutional analysis. J Heart Lung Transplant 2012; 31:67–72.
- Drazner MH, Velez-Martinez M, Ayers CR, et al. Relationship of right- to left-sided ventricular filling pressures in advanced heart failure: insights from the ESCAPE trial. Circ Heart Fail 2013; 6:264–270.
- Grodin JL, Drazner MH, Dupont M, et al. A disproportionate elevation in right ventricular filling pressure, in relation to left ventricular filling pressure, is associated with renal impairment and increased mortality in advanced decompensated heart failure. Am Heart J 2015; 169:806–812.
- Bart BA, Goldsmith SR, Lee KL, et al, for the Heart Failure Clinical Research Network. Ultrafiltration in decompensated heart failure with cardiorenal syndrome. N Engl J Med 2012; 367:2296–2304.
- Chen HH, Anstrom KJ, Givertz MM, et al; NHLBI Heart Failure Clinical Research Network. Low-dose dopamine or low-dose nesiritide in acute heart failure with renal dysfunction: the ROSE acute heart failure randomized trial. JAMA 2013; 310:2533–2543.
- Gorodeski EZ, Chu EC, Reese JR, Shishehbor MH, Hsich E, Starling RC. Prognosis on chronic dobutamine or milrinone infusions for stage D heart failure. Circ Heart Fail 2009; 2:320–324.
- Cuffe MS, Califf RM, Adams KF Jr, et al, for the Outcomes of a Prospective Trial of Intravenous Milrinone for Exacerbations of Chronic Heart Failure (OPTIME-CHF) Investigators. Short-term intravenous milrinone for acute exacerbation of chronic heart failure: a randomized controlled trial. JAMA 2002; 287:1541–1547.
- Massie BM, O’Connor CM, Metra M, et al, for the PROTECT Investigators and Committees. Rolofylline, an adenosine A1-receptor antagonist, in acute heart failure. N Engl J Med 2010; 363:1419–1428.
Having the COURAGE to include PCI in shared decision-making for stable angina
Invented by Andreas Grüntzig in 1977, percutaneous coronary intervention (PCI) has revolutionized the management of coronary artery disease.1 Initially, PCI was more attractive than conventional revascularization with coronary artery bypass grafting because it was less invasive, but as time went on PCI acquired its own evidence base of improved clinical outcomes. In fact, for ST-elevation myocardial infarction, non-ST-elevation myocardial infarction, and cardiogenic shock, there is clear evidence that PCI saves lives in both the short and long term.2,3
But PCI is also used widely in stable coronary artery disease, and in contrast to the clear-cut benefit in the acute conditions noted above, a series of reports culminating in the Clinical Outcomes Utilizing Revascularization and Aggressive Drug Evaluation (COURAGE) trial has shown that PCI in stable coronary artery disease does not reduce the risk of death or of subsequent myocardial infarction.4,5 Cardiologists have heeded the COURAGE trial findings in their clinical decision-making, and the rate of PCI for stable coronary artery disease dropped by 60% from 2006 to 2011.6
In an article in this issue,7 Dr. Michael Rothberg describes a 55-year-old man who develops new-onset angina and then undergoes a Bruce protocol stress test that is stopped at 6 minutes due to chest pain and ST-segment depression. Dr. Rothberg argues that, based on COURAGE trial data, this patient and other patients with stable coronary artery disease should not be treated with PCI but instead should receive optimal medical therapy.
KEY ISSUES ABOUT THE COURAGE TRIAL
To understand the applicability of the results of the COURAGE trial to patient care, it is important to examine a number of key issues about this trial.
First, COURAGE enrolled a narrow group of patients with stable coronary artery disease and excluded many common patient subgroups, such as those with heart failure, severe anginal symptoms, or left main artery stenosis, who would benefit from revascularization.5 Specifically, for every 100 patients enrolled in COURAGE, 161 were excluded for having heart failure, 39 were excluded for class IV angina, and 31 were excluded for left main stenosis.
Second, although COURAGE has been described as a trial of PCI vs optimal medical therapy, it was not. Rather, it was a trial of optimal medical therapy with PCI first vs optimal medical therapy with crossover PCI if medical therapy failed.5 The crossover rate was not insubstantial: 16.1% of the patients in the medical therapy group underwent PCI by the end of the first year, increasing to 32.6% at a median of 4.6 years of follow-up.5,7 And patients with more intense and frequent angina and resulting worse quality of life were the ones who required crossover PCI.8
Third, it has been proposed that patients with suspicious cardiac symptoms or abnormal stress test findings can be managed with optimal medical therapy initially, based on the COURAGE findings. However, the COURAGE trial required diagnostic angiography both to confirm underlying coronary artery disease and to exclude left main disease.5 Thus, regardless of one’s position on the role of PCI in stable coronary artery disease, diagnostic investigation by cardiac catheterization or computed tomographic angiography to confirm the presence or absence of coronary artery disease remains mandatory.
Fourth, optimal medical therapy was prescribed by the trial’s protocol, so that one would expect that both treatment groups received similar levels of optimal medical therapy. However, the optimal medical therapy group required more medications to achieve the same outcome as the PCI group.5
Finally, although it has been reported that the COURAGE trial showed no benefit for PCI, in fact, for the outcome of symptom relief, initial PCI was clearly superior to optimal medical therapy beginning at 3 months and extending out to 24 months—a result for which the magnitude of benefit is underestimated due to the occurrence of crossover PCI.9 In particular, women and patients with a high frequency of angina derived improvement in angina-related quality of life from PCI compared with optimal medical therapy.8,10
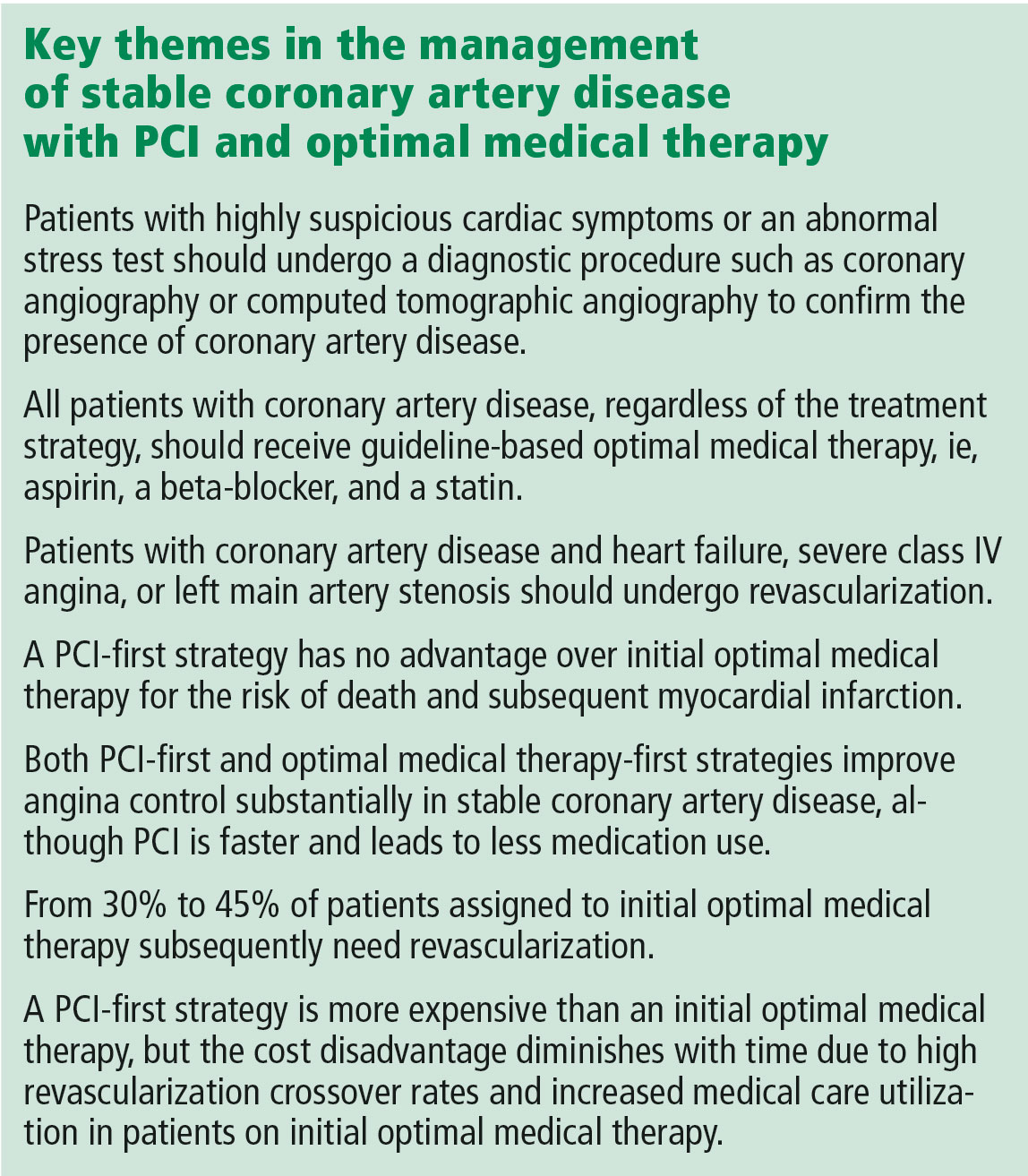
A MORE NUANCED INTERPRETATION
For these reasons, the role of PCI in stable coronary disease is more nuanced than simply stating that the COURAGE trial results were “negative” for PCI. It is more accurate to say that in selected patients with moderate symptoms of angina and without heart failure or left main artery disease, a PCI-first strategy has no advantage over an optimal medical treatment-first strategy for the risk of death and myocardial infarction but does lead to earlier angina relief and less long-term need for medication. In addition, in up to one-third of cases, an optimal medical treatment-first strategy fails and requires crossover to PCI.5
Dr. Rothberg is correct in highlighting the crucial importance of optimal medical therapy in the management of stable coronary artery disease. In fact, cardiologists strive to prescribe optimal medical treatment for all coronary artery disease patients irrespective of treatment strategy. However, 3 important issues in his analysis need to be highlighted.
Controlling symptoms is important, and we should not underrate it. The patient described in Dr. Rothberg’s article could exercise for only 6 minutes on a Bruce treadmill test, indicating a quite limited functional capacity of only 5.8 metabolic equivalents of the task (METs).11 (A healthy 55-year-old man should be able to achieve 10.5 METs.12) Inability to achieve 6 METs precludes the ability to dance, to ride a bike at a moderate pace, or to go on a hike.13 For many patients, these limitations are serious and important concerns for their lifestyle and quality of life. PCI has been shown to be superior to medical therapy in improving functional capacity, improving it by 20% vs 2% in one trial14 and 26% vs 7% in a second trial.14 Patients undergoing PCI were twice as likely to have a greater than 2-minute increase in exercise capacity.15 Recognizing the importance of symptom control in stable coronary artery disease is patient-centered care.
Patient decision-making is complicated, and we should not assume that patients choose PCI primarily to reduce their risk of death. A randomized trial showed that patients continued to select PCI as initial treatment even when they clearly knew that it would not prevent death or myocardial infarction.16 As noted above, patients may value earlier symptom relief, particularly if their angina is frequent or limiting. In addition, patients strongly desire to minimize medical therapy and may be willing to trade decreased life expectancy to reduce the need to take medications.17 Finally, some patients may want to be able to continue to participate in certain lifestyle activities.
PCI is expensive, but less so over the long run. With a PCI-first strategy, costs are front-loaded, and studies with short-term follow-up show a marked increase in cost. However, long-term follow-up shows that the cost differences diminish dramatically due to high rates of crossover to revascularization and increased medical care in the optimal medical therapy arm. The cumulative lifetime costs in the COURAGE trial with a PCI-first strategy, although statistically significant, were only 10% higher than with the optimal medical treatment-first strategy ($99,820 vs $90,370).18 Therefore, substantial long-term cost-savings by shifting from an initial PCI strategy to initial optimal medical therapy are unlikely to be delivered when measured over the long term.
NEWER TRIALS SUPPORT A BALANCED APPROACH
The most recent studies of the management of stable coronary artery disease support a balanced approach.
The ORBITA trial (Objective Randomised Blinded Investigation With Optimal Medical Therapy of Angioplasty in Stable Angina), on one hand, showed limited benefit of PCI vs medical therapy in patients with single-vessel coronary artery disease, preserved functional capacity, and mild symptoms.19 There was no significant improvement in exercise capacity or angina frequency, although baseline angina frequency after medical stabilization was quite low.
The FAME 2 trial (Fractional Flow Reserve Versus Angiography for Multivessel Evaluation), on the other hand, studied patients with positive fractional flow reserve coronary artery disease (ie, using an invasive technique to confirm the hemodynamic significance of the coronary stenosis) and showed markedly better outcomes with PCI than with medical therapy.20 Specifically, the PCI-first group had improved quality of life and dramatically less need for urgent revascularization.
Furthermore, as in the COURAGE trial, the optimal medical therapy group had a high crossover rate to PCI (44.2%), leading to the complete elimination of the early cost advantage of medical therapy by 3 years. The initial costs with PCI vs medical therapy were $9,944 vs $4,439 (P < .001); the 3-year costs were $16,792 vs $16,737 (P = .94).
For these reasons, a balanced approach to recommending PCI first vs optimal medical treatment first remains the best strategy.
TOWARD PATIENT-CENTERED CARE
For the 55-year-old patient in Dr. Rothberg’s article, the first step in making an appropriate decision would be to understand the severity of symptoms relative to the patient’s lifestyle. The second step is to assess the patient’s interest in an invasive procedure such as PCI relative to optimal medical therapy, as the patient may have a strong preference for one option or the other.
Finally, with the understanding that there is no difference in hard end points of myocardial infarction and death, a balanced discussion of the advantages and disadvantages of both PCI and optimal medical therapy would be needed. For PCI, advantages include earlier symptom control and improved quality of the life, particularly if symptoms are severe, with disadvantages of an invasive procedure with its attendant risks. For optimal medical therapy, advantages include improved symptom control and avoidance of an invasive procedure, while disadvantages include increased medication use and a high rate of eventual crossover to PCI. This important discussion integrating both patient and medical perspectives ultimately leads to the best decision for the individual patient.
A patient-centered approach to clinical decision-making mandates inclusion of PCI first as an option in the management of stable coronary artery disease. After confirming the patient has coronary artery disease, patients with heart failure, class IV angina at rest, or left main artery stenosis should be referred for revascularization. In the remaining patients with confirmed coronary artery disease and moderate angina symptoms, either PCI first or optimal medical therapy first is an appropriate initial strategy that considers coronary anatomy, symptom burden, and patient desires.
- Meier B. The first patient to undergo coronary angioplasty—23-year follow-up. N Engl J Med 2001; 344:144–145.
- Levine GN, Bates ER, Blankenship JC, et al. 2011 ACCF/AHA/SCAI Guideline for Percutaneous Coronary Intervention: executive summary: a report of the American College of Cardiology Foundation/American Heart Association Task Force on Practice Guidelines and the Society for Cardiovascular Angiography and Interventions. Circulation 2011; 124:2574–2609.
- Fox KAA, Clayton TC, Damman P, et al. Long-term outcome of a routine versus selective invasive strategy in patients with non-ST-segment elevation acute coronary syndrome a meta-analysis of individual patient data. J Am Coll Cardiol 2010; 55:2435–2445.
- Katritsis DG, Ioannidis JPA. Percutaneous coronary intervention versus conservative therapy in nonacute coronary artery disease: a meta-analysis. Circulation 2005; 111:2906–2912.
- Boden WE, O’Rourke RA, Teo KK, et al. Optimal medical therapy with or without PCI for stable coronary disease. N Engl J Med 2007; 356:1503–1516.
- Bangalore S, Gupta N, Généreux P, Guo Y, Pancholy S, Feit F. Trend in percutaneous coronary intervention volume following the COURAGE and BARI-2D trials: insight from over 8.1 million percutaneous coronary interventions. Int J Cardiol 2015; 183:6–10.
- Rothberg MB. PCI for stable angina: a missed opportunity for shared decision-making. Cleve Clin J Med 2018; 85:105–121.
- Spertus JA, Maron DJ, Cohen DJ, et al. Frequency, predictors, and consequences of crossing over to revascularization within 12 months of randomization to optimal medical therapy in the Clinical Outcomes Utilizing Revascularization and Aggressive Drug Evaluation (COURAGE) trial. Circ Cardiovasc Qual Outcomes 2013; 6:409–418.
- Weintraub WS, Spertus JA, Kolm P, et al. Effect of PCI on quality of life in patients with stable coronary disease. N Engl J Med 2008; 359:677–687.
- Acharjee S, Teo KK, Jacobs AK, et al. Optimal medical therapy with or without percutaneous coronary intervention in women with stable coronary disease: a pre-specified subset analysis of the Clinical Outcomes Utilizing Revascularization and Aggressive druG Evaluation (COURAGE) trial. Am Heart J 2016; 173:108–117.
- Foster C, Jackson AS, Pollock ML, et al. Generalized equations for predicting functional capacity from treadmill performance. Am Heart J 1984; 107:1229–1234.
- Morris CK, Myers J, Froelicher VF, Kawaguchi T, Ueshima K, Hideg A. Nomogram based on metabolic equivalents and age for assessing aerobic exercise capacity in men. J Am Coll Cardiol 1993; 22:175–182.
- Jetté M, Sidney K, Blümchen G. Metabolic equivalents (METS) in exercise testing, exercise prescription, and evaluation of functional capacity. Clin Cardiol 1990; 13:555–565.
- Erne P, Schoenenberger AW, Burckhardt D, et al. Effects of percutaneous coronary interventions in silent ischemia after myocardial infarction: the SWISSI II randomized controlled trial. JAMA 2007; 297:1985–1991.
- Strauss WE, Fortin T, Hartigan P, Folland ED, Parisi AF. A comparison of quality of life scores in patients with angina pectoris after angioplasty compared with after medical therapy. Outcomes of a randomized clinical trial. Veterans Affairs Study of Angioplasty Compared to Medical Therapy Investigators. Circulation 1995; 92:1710–1719.
- Coylewright M, Dick S, Zmolek B, et al. PCI choice decision aid for stable coronary artery disease: a randomized trial. Circ Cardiovasc Qual Outcomes 2016; 9:767–776.
- Fontana M, Asaria P, Moraldo M, et al. Patient-accessible tool for shared decision making in cardiovascular primary prevention: balancing longevity benefits against medication disutility. Circulation 2014; 129:2539–2546.
- Weintraub WS, Boden WE, Zhang Z, et al. Cost-effectiveness of percutaneous coronary intervention in optimally treated stable coronary patients. Circ Cardiovasc Qual Outcomes 2008; 1:12–20.
- Al-Lamee R, Thompson D, Dehbi H-M, et al. Percutaneous coronary intervention in stable angina (ORBITA): a double-blind, randomised controlled trial. Lancet November 2, 2017; doi:10.1016/S0140-6736(17)32714-9.
- Fearon WF, Nishi T, Bruyne BD, et al. Clinical outcomes and cost-effectiveness of fractional flow reserve-guided percutaneous coronary intervention in patients with stable coronary artery disease: three-year follow-up of the FAME 2 Trial (Fractional Flow Reserve Versus Angiography for Multivessel Evaluation). Circulation November 2017; doi:10.1161/CIRCULATIONAHA.117.031907.
Invented by Andreas Grüntzig in 1977, percutaneous coronary intervention (PCI) has revolutionized the management of coronary artery disease.1 Initially, PCI was more attractive than conventional revascularization with coronary artery bypass grafting because it was less invasive, but as time went on PCI acquired its own evidence base of improved clinical outcomes. In fact, for ST-elevation myocardial infarction, non-ST-elevation myocardial infarction, and cardiogenic shock, there is clear evidence that PCI saves lives in both the short and long term.2,3
But PCI is also used widely in stable coronary artery disease, and in contrast to the clear-cut benefit in the acute conditions noted above, a series of reports culminating in the Clinical Outcomes Utilizing Revascularization and Aggressive Drug Evaluation (COURAGE) trial has shown that PCI in stable coronary artery disease does not reduce the risk of death or of subsequent myocardial infarction.4,5 Cardiologists have heeded the COURAGE trial findings in their clinical decision-making, and the rate of PCI for stable coronary artery disease dropped by 60% from 2006 to 2011.6
In an article in this issue,7 Dr. Michael Rothberg describes a 55-year-old man who develops new-onset angina and then undergoes a Bruce protocol stress test that is stopped at 6 minutes due to chest pain and ST-segment depression. Dr. Rothberg argues that, based on COURAGE trial data, this patient and other patients with stable coronary artery disease should not be treated with PCI but instead should receive optimal medical therapy.
KEY ISSUES ABOUT THE COURAGE TRIAL
To understand the applicability of the results of the COURAGE trial to patient care, it is important to examine a number of key issues about this trial.
First, COURAGE enrolled a narrow group of patients with stable coronary artery disease and excluded many common patient subgroups, such as those with heart failure, severe anginal symptoms, or left main artery stenosis, who would benefit from revascularization.5 Specifically, for every 100 patients enrolled in COURAGE, 161 were excluded for having heart failure, 39 were excluded for class IV angina, and 31 were excluded for left main stenosis.
Second, although COURAGE has been described as a trial of PCI vs optimal medical therapy, it was not. Rather, it was a trial of optimal medical therapy with PCI first vs optimal medical therapy with crossover PCI if medical therapy failed.5 The crossover rate was not insubstantial: 16.1% of the patients in the medical therapy group underwent PCI by the end of the first year, increasing to 32.6% at a median of 4.6 years of follow-up.5,7 And patients with more intense and frequent angina and resulting worse quality of life were the ones who required crossover PCI.8
Third, it has been proposed that patients with suspicious cardiac symptoms or abnormal stress test findings can be managed with optimal medical therapy initially, based on the COURAGE findings. However, the COURAGE trial required diagnostic angiography both to confirm underlying coronary artery disease and to exclude left main disease.5 Thus, regardless of one’s position on the role of PCI in stable coronary artery disease, diagnostic investigation by cardiac catheterization or computed tomographic angiography to confirm the presence or absence of coronary artery disease remains mandatory.
Fourth, optimal medical therapy was prescribed by the trial’s protocol, so that one would expect that both treatment groups received similar levels of optimal medical therapy. However, the optimal medical therapy group required more medications to achieve the same outcome as the PCI group.5
Finally, although it has been reported that the COURAGE trial showed no benefit for PCI, in fact, for the outcome of symptom relief, initial PCI was clearly superior to optimal medical therapy beginning at 3 months and extending out to 24 months—a result for which the magnitude of benefit is underestimated due to the occurrence of crossover PCI.9 In particular, women and patients with a high frequency of angina derived improvement in angina-related quality of life from PCI compared with optimal medical therapy.8,10
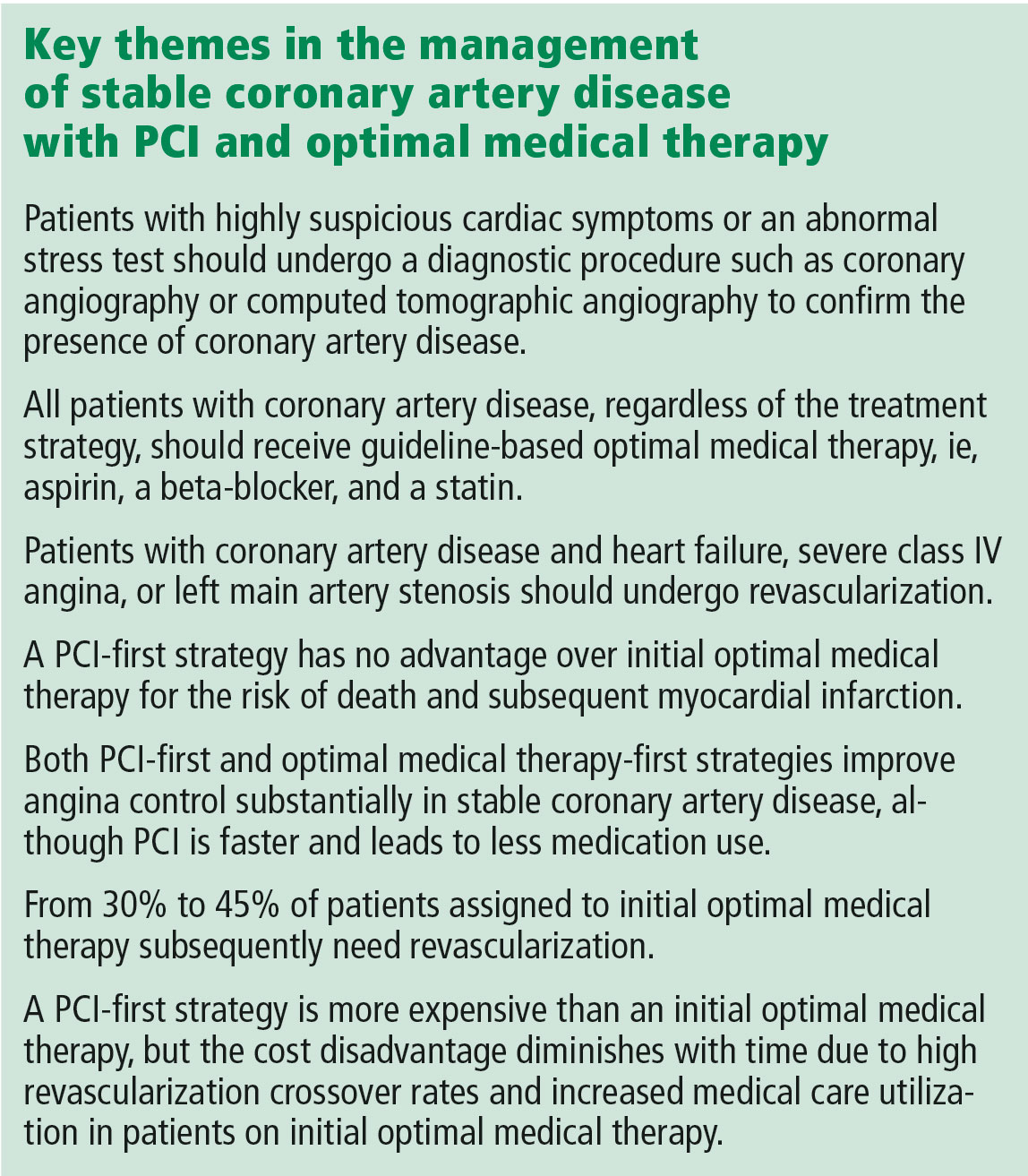
A MORE NUANCED INTERPRETATION
For these reasons, the role of PCI in stable coronary disease is more nuanced than simply stating that the COURAGE trial results were “negative” for PCI. It is more accurate to say that in selected patients with moderate symptoms of angina and without heart failure or left main artery disease, a PCI-first strategy has no advantage over an optimal medical treatment-first strategy for the risk of death and myocardial infarction but does lead to earlier angina relief and less long-term need for medication. In addition, in up to one-third of cases, an optimal medical treatment-first strategy fails and requires crossover to PCI.5
Dr. Rothberg is correct in highlighting the crucial importance of optimal medical therapy in the management of stable coronary artery disease. In fact, cardiologists strive to prescribe optimal medical treatment for all coronary artery disease patients irrespective of treatment strategy. However, 3 important issues in his analysis need to be highlighted.
Controlling symptoms is important, and we should not underrate it. The patient described in Dr. Rothberg’s article could exercise for only 6 minutes on a Bruce treadmill test, indicating a quite limited functional capacity of only 5.8 metabolic equivalents of the task (METs).11 (A healthy 55-year-old man should be able to achieve 10.5 METs.12) Inability to achieve 6 METs precludes the ability to dance, to ride a bike at a moderate pace, or to go on a hike.13 For many patients, these limitations are serious and important concerns for their lifestyle and quality of life. PCI has been shown to be superior to medical therapy in improving functional capacity, improving it by 20% vs 2% in one trial14 and 26% vs 7% in a second trial.14 Patients undergoing PCI were twice as likely to have a greater than 2-minute increase in exercise capacity.15 Recognizing the importance of symptom control in stable coronary artery disease is patient-centered care.
Patient decision-making is complicated, and we should not assume that patients choose PCI primarily to reduce their risk of death. A randomized trial showed that patients continued to select PCI as initial treatment even when they clearly knew that it would not prevent death or myocardial infarction.16 As noted above, patients may value earlier symptom relief, particularly if their angina is frequent or limiting. In addition, patients strongly desire to minimize medical therapy and may be willing to trade decreased life expectancy to reduce the need to take medications.17 Finally, some patients may want to be able to continue to participate in certain lifestyle activities.
PCI is expensive, but less so over the long run. With a PCI-first strategy, costs are front-loaded, and studies with short-term follow-up show a marked increase in cost. However, long-term follow-up shows that the cost differences diminish dramatically due to high rates of crossover to revascularization and increased medical care in the optimal medical therapy arm. The cumulative lifetime costs in the COURAGE trial with a PCI-first strategy, although statistically significant, were only 10% higher than with the optimal medical treatment-first strategy ($99,820 vs $90,370).18 Therefore, substantial long-term cost-savings by shifting from an initial PCI strategy to initial optimal medical therapy are unlikely to be delivered when measured over the long term.
NEWER TRIALS SUPPORT A BALANCED APPROACH
The most recent studies of the management of stable coronary artery disease support a balanced approach.
The ORBITA trial (Objective Randomised Blinded Investigation With Optimal Medical Therapy of Angioplasty in Stable Angina), on one hand, showed limited benefit of PCI vs medical therapy in patients with single-vessel coronary artery disease, preserved functional capacity, and mild symptoms.19 There was no significant improvement in exercise capacity or angina frequency, although baseline angina frequency after medical stabilization was quite low.
The FAME 2 trial (Fractional Flow Reserve Versus Angiography for Multivessel Evaluation), on the other hand, studied patients with positive fractional flow reserve coronary artery disease (ie, using an invasive technique to confirm the hemodynamic significance of the coronary stenosis) and showed markedly better outcomes with PCI than with medical therapy.20 Specifically, the PCI-first group had improved quality of life and dramatically less need for urgent revascularization.
Furthermore, as in the COURAGE trial, the optimal medical therapy group had a high crossover rate to PCI (44.2%), leading to the complete elimination of the early cost advantage of medical therapy by 3 years. The initial costs with PCI vs medical therapy were $9,944 vs $4,439 (P < .001); the 3-year costs were $16,792 vs $16,737 (P = .94).
For these reasons, a balanced approach to recommending PCI first vs optimal medical treatment first remains the best strategy.
TOWARD PATIENT-CENTERED CARE
For the 55-year-old patient in Dr. Rothberg’s article, the first step in making an appropriate decision would be to understand the severity of symptoms relative to the patient’s lifestyle. The second step is to assess the patient’s interest in an invasive procedure such as PCI relative to optimal medical therapy, as the patient may have a strong preference for one option or the other.
Finally, with the understanding that there is no difference in hard end points of myocardial infarction and death, a balanced discussion of the advantages and disadvantages of both PCI and optimal medical therapy would be needed. For PCI, advantages include earlier symptom control and improved quality of the life, particularly if symptoms are severe, with disadvantages of an invasive procedure with its attendant risks. For optimal medical therapy, advantages include improved symptom control and avoidance of an invasive procedure, while disadvantages include increased medication use and a high rate of eventual crossover to PCI. This important discussion integrating both patient and medical perspectives ultimately leads to the best decision for the individual patient.
A patient-centered approach to clinical decision-making mandates inclusion of PCI first as an option in the management of stable coronary artery disease. After confirming the patient has coronary artery disease, patients with heart failure, class IV angina at rest, or left main artery stenosis should be referred for revascularization. In the remaining patients with confirmed coronary artery disease and moderate angina symptoms, either PCI first or optimal medical therapy first is an appropriate initial strategy that considers coronary anatomy, symptom burden, and patient desires.
Invented by Andreas Grüntzig in 1977, percutaneous coronary intervention (PCI) has revolutionized the management of coronary artery disease.1 Initially, PCI was more attractive than conventional revascularization with coronary artery bypass grafting because it was less invasive, but as time went on PCI acquired its own evidence base of improved clinical outcomes. In fact, for ST-elevation myocardial infarction, non-ST-elevation myocardial infarction, and cardiogenic shock, there is clear evidence that PCI saves lives in both the short and long term.2,3
But PCI is also used widely in stable coronary artery disease, and in contrast to the clear-cut benefit in the acute conditions noted above, a series of reports culminating in the Clinical Outcomes Utilizing Revascularization and Aggressive Drug Evaluation (COURAGE) trial has shown that PCI in stable coronary artery disease does not reduce the risk of death or of subsequent myocardial infarction.4,5 Cardiologists have heeded the COURAGE trial findings in their clinical decision-making, and the rate of PCI for stable coronary artery disease dropped by 60% from 2006 to 2011.6
In an article in this issue,7 Dr. Michael Rothberg describes a 55-year-old man who develops new-onset angina and then undergoes a Bruce protocol stress test that is stopped at 6 minutes due to chest pain and ST-segment depression. Dr. Rothberg argues that, based on COURAGE trial data, this patient and other patients with stable coronary artery disease should not be treated with PCI but instead should receive optimal medical therapy.
KEY ISSUES ABOUT THE COURAGE TRIAL
To understand the applicability of the results of the COURAGE trial to patient care, it is important to examine a number of key issues about this trial.
First, COURAGE enrolled a narrow group of patients with stable coronary artery disease and excluded many common patient subgroups, such as those with heart failure, severe anginal symptoms, or left main artery stenosis, who would benefit from revascularization.5 Specifically, for every 100 patients enrolled in COURAGE, 161 were excluded for having heart failure, 39 were excluded for class IV angina, and 31 were excluded for left main stenosis.
Second, although COURAGE has been described as a trial of PCI vs optimal medical therapy, it was not. Rather, it was a trial of optimal medical therapy with PCI first vs optimal medical therapy with crossover PCI if medical therapy failed.5 The crossover rate was not insubstantial: 16.1% of the patients in the medical therapy group underwent PCI by the end of the first year, increasing to 32.6% at a median of 4.6 years of follow-up.5,7 And patients with more intense and frequent angina and resulting worse quality of life were the ones who required crossover PCI.8
Third, it has been proposed that patients with suspicious cardiac symptoms or abnormal stress test findings can be managed with optimal medical therapy initially, based on the COURAGE findings. However, the COURAGE trial required diagnostic angiography both to confirm underlying coronary artery disease and to exclude left main disease.5 Thus, regardless of one’s position on the role of PCI in stable coronary artery disease, diagnostic investigation by cardiac catheterization or computed tomographic angiography to confirm the presence or absence of coronary artery disease remains mandatory.
Fourth, optimal medical therapy was prescribed by the trial’s protocol, so that one would expect that both treatment groups received similar levels of optimal medical therapy. However, the optimal medical therapy group required more medications to achieve the same outcome as the PCI group.5
Finally, although it has been reported that the COURAGE trial showed no benefit for PCI, in fact, for the outcome of symptom relief, initial PCI was clearly superior to optimal medical therapy beginning at 3 months and extending out to 24 months—a result for which the magnitude of benefit is underestimated due to the occurrence of crossover PCI.9 In particular, women and patients with a high frequency of angina derived improvement in angina-related quality of life from PCI compared with optimal medical therapy.8,10
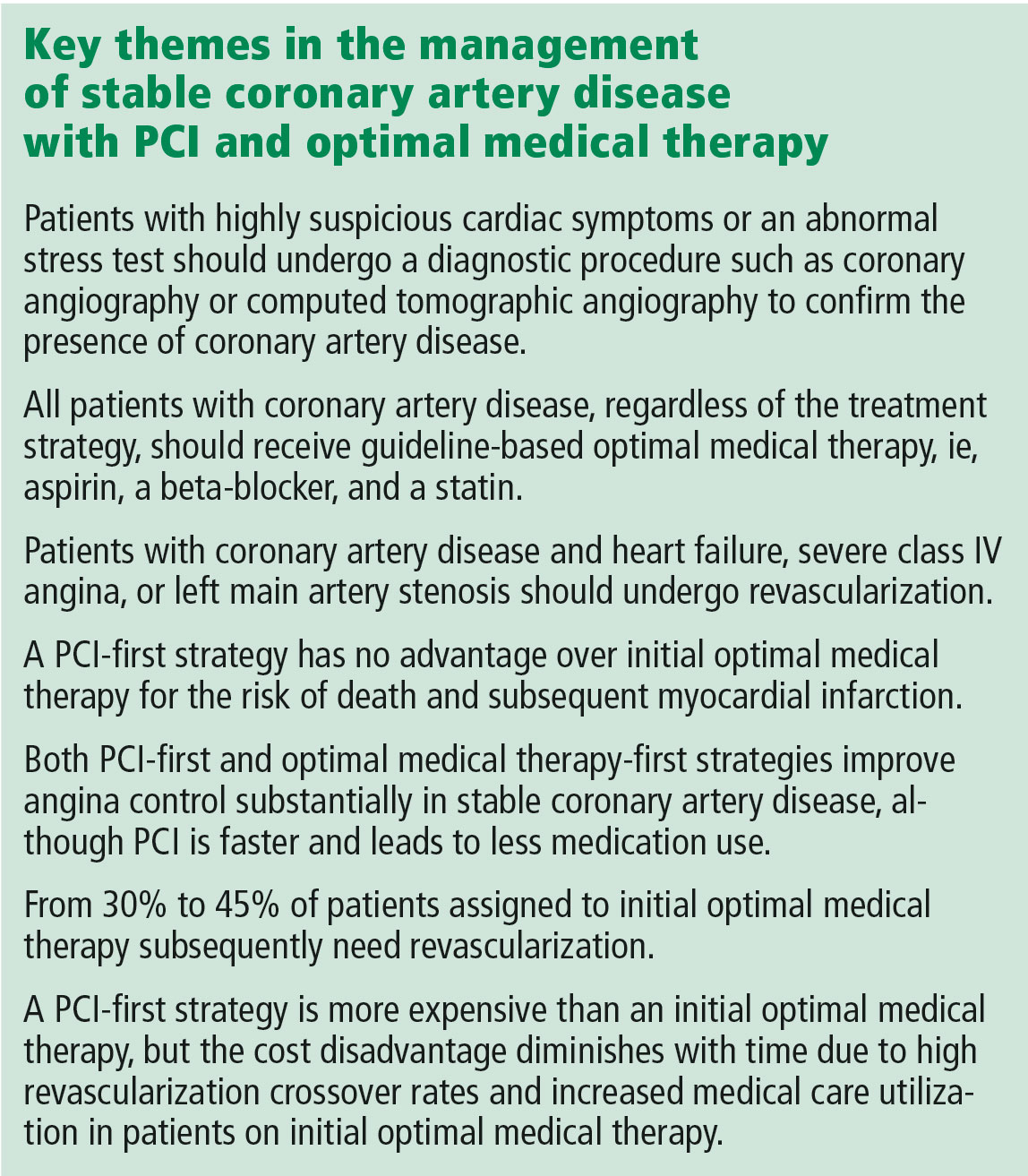
A MORE NUANCED INTERPRETATION
For these reasons, the role of PCI in stable coronary disease is more nuanced than simply stating that the COURAGE trial results were “negative” for PCI. It is more accurate to say that in selected patients with moderate symptoms of angina and without heart failure or left main artery disease, a PCI-first strategy has no advantage over an optimal medical treatment-first strategy for the risk of death and myocardial infarction but does lead to earlier angina relief and less long-term need for medication. In addition, in up to one-third of cases, an optimal medical treatment-first strategy fails and requires crossover to PCI.5
Dr. Rothberg is correct in highlighting the crucial importance of optimal medical therapy in the management of stable coronary artery disease. In fact, cardiologists strive to prescribe optimal medical treatment for all coronary artery disease patients irrespective of treatment strategy. However, 3 important issues in his analysis need to be highlighted.
Controlling symptoms is important, and we should not underrate it. The patient described in Dr. Rothberg’s article could exercise for only 6 minutes on a Bruce treadmill test, indicating a quite limited functional capacity of only 5.8 metabolic equivalents of the task (METs).11 (A healthy 55-year-old man should be able to achieve 10.5 METs.12) Inability to achieve 6 METs precludes the ability to dance, to ride a bike at a moderate pace, or to go on a hike.13 For many patients, these limitations are serious and important concerns for their lifestyle and quality of life. PCI has been shown to be superior to medical therapy in improving functional capacity, improving it by 20% vs 2% in one trial14 and 26% vs 7% in a second trial.14 Patients undergoing PCI were twice as likely to have a greater than 2-minute increase in exercise capacity.15 Recognizing the importance of symptom control in stable coronary artery disease is patient-centered care.
Patient decision-making is complicated, and we should not assume that patients choose PCI primarily to reduce their risk of death. A randomized trial showed that patients continued to select PCI as initial treatment even when they clearly knew that it would not prevent death or myocardial infarction.16 As noted above, patients may value earlier symptom relief, particularly if their angina is frequent or limiting. In addition, patients strongly desire to minimize medical therapy and may be willing to trade decreased life expectancy to reduce the need to take medications.17 Finally, some patients may want to be able to continue to participate in certain lifestyle activities.
PCI is expensive, but less so over the long run. With a PCI-first strategy, costs are front-loaded, and studies with short-term follow-up show a marked increase in cost. However, long-term follow-up shows that the cost differences diminish dramatically due to high rates of crossover to revascularization and increased medical care in the optimal medical therapy arm. The cumulative lifetime costs in the COURAGE trial with a PCI-first strategy, although statistically significant, were only 10% higher than with the optimal medical treatment-first strategy ($99,820 vs $90,370).18 Therefore, substantial long-term cost-savings by shifting from an initial PCI strategy to initial optimal medical therapy are unlikely to be delivered when measured over the long term.
NEWER TRIALS SUPPORT A BALANCED APPROACH
The most recent studies of the management of stable coronary artery disease support a balanced approach.
The ORBITA trial (Objective Randomised Blinded Investigation With Optimal Medical Therapy of Angioplasty in Stable Angina), on one hand, showed limited benefit of PCI vs medical therapy in patients with single-vessel coronary artery disease, preserved functional capacity, and mild symptoms.19 There was no significant improvement in exercise capacity or angina frequency, although baseline angina frequency after medical stabilization was quite low.
The FAME 2 trial (Fractional Flow Reserve Versus Angiography for Multivessel Evaluation), on the other hand, studied patients with positive fractional flow reserve coronary artery disease (ie, using an invasive technique to confirm the hemodynamic significance of the coronary stenosis) and showed markedly better outcomes with PCI than with medical therapy.20 Specifically, the PCI-first group had improved quality of life and dramatically less need for urgent revascularization.
Furthermore, as in the COURAGE trial, the optimal medical therapy group had a high crossover rate to PCI (44.2%), leading to the complete elimination of the early cost advantage of medical therapy by 3 years. The initial costs with PCI vs medical therapy were $9,944 vs $4,439 (P < .001); the 3-year costs were $16,792 vs $16,737 (P = .94).
For these reasons, a balanced approach to recommending PCI first vs optimal medical treatment first remains the best strategy.
TOWARD PATIENT-CENTERED CARE
For the 55-year-old patient in Dr. Rothberg’s article, the first step in making an appropriate decision would be to understand the severity of symptoms relative to the patient’s lifestyle. The second step is to assess the patient’s interest in an invasive procedure such as PCI relative to optimal medical therapy, as the patient may have a strong preference for one option or the other.
Finally, with the understanding that there is no difference in hard end points of myocardial infarction and death, a balanced discussion of the advantages and disadvantages of both PCI and optimal medical therapy would be needed. For PCI, advantages include earlier symptom control and improved quality of the life, particularly if symptoms are severe, with disadvantages of an invasive procedure with its attendant risks. For optimal medical therapy, advantages include improved symptom control and avoidance of an invasive procedure, while disadvantages include increased medication use and a high rate of eventual crossover to PCI. This important discussion integrating both patient and medical perspectives ultimately leads to the best decision for the individual patient.
A patient-centered approach to clinical decision-making mandates inclusion of PCI first as an option in the management of stable coronary artery disease. After confirming the patient has coronary artery disease, patients with heart failure, class IV angina at rest, or left main artery stenosis should be referred for revascularization. In the remaining patients with confirmed coronary artery disease and moderate angina symptoms, either PCI first or optimal medical therapy first is an appropriate initial strategy that considers coronary anatomy, symptom burden, and patient desires.
- Meier B. The first patient to undergo coronary angioplasty—23-year follow-up. N Engl J Med 2001; 344:144–145.
- Levine GN, Bates ER, Blankenship JC, et al. 2011 ACCF/AHA/SCAI Guideline for Percutaneous Coronary Intervention: executive summary: a report of the American College of Cardiology Foundation/American Heart Association Task Force on Practice Guidelines and the Society for Cardiovascular Angiography and Interventions. Circulation 2011; 124:2574–2609.
- Fox KAA, Clayton TC, Damman P, et al. Long-term outcome of a routine versus selective invasive strategy in patients with non-ST-segment elevation acute coronary syndrome a meta-analysis of individual patient data. J Am Coll Cardiol 2010; 55:2435–2445.
- Katritsis DG, Ioannidis JPA. Percutaneous coronary intervention versus conservative therapy in nonacute coronary artery disease: a meta-analysis. Circulation 2005; 111:2906–2912.
- Boden WE, O’Rourke RA, Teo KK, et al. Optimal medical therapy with or without PCI for stable coronary disease. N Engl J Med 2007; 356:1503–1516.
- Bangalore S, Gupta N, Généreux P, Guo Y, Pancholy S, Feit F. Trend in percutaneous coronary intervention volume following the COURAGE and BARI-2D trials: insight from over 8.1 million percutaneous coronary interventions. Int J Cardiol 2015; 183:6–10.
- Rothberg MB. PCI for stable angina: a missed opportunity for shared decision-making. Cleve Clin J Med 2018; 85:105–121.
- Spertus JA, Maron DJ, Cohen DJ, et al. Frequency, predictors, and consequences of crossing over to revascularization within 12 months of randomization to optimal medical therapy in the Clinical Outcomes Utilizing Revascularization and Aggressive Drug Evaluation (COURAGE) trial. Circ Cardiovasc Qual Outcomes 2013; 6:409–418.
- Weintraub WS, Spertus JA, Kolm P, et al. Effect of PCI on quality of life in patients with stable coronary disease. N Engl J Med 2008; 359:677–687.
- Acharjee S, Teo KK, Jacobs AK, et al. Optimal medical therapy with or without percutaneous coronary intervention in women with stable coronary disease: a pre-specified subset analysis of the Clinical Outcomes Utilizing Revascularization and Aggressive druG Evaluation (COURAGE) trial. Am Heart J 2016; 173:108–117.
- Foster C, Jackson AS, Pollock ML, et al. Generalized equations for predicting functional capacity from treadmill performance. Am Heart J 1984; 107:1229–1234.
- Morris CK, Myers J, Froelicher VF, Kawaguchi T, Ueshima K, Hideg A. Nomogram based on metabolic equivalents and age for assessing aerobic exercise capacity in men. J Am Coll Cardiol 1993; 22:175–182.
- Jetté M, Sidney K, Blümchen G. Metabolic equivalents (METS) in exercise testing, exercise prescription, and evaluation of functional capacity. Clin Cardiol 1990; 13:555–565.
- Erne P, Schoenenberger AW, Burckhardt D, et al. Effects of percutaneous coronary interventions in silent ischemia after myocardial infarction: the SWISSI II randomized controlled trial. JAMA 2007; 297:1985–1991.
- Strauss WE, Fortin T, Hartigan P, Folland ED, Parisi AF. A comparison of quality of life scores in patients with angina pectoris after angioplasty compared with after medical therapy. Outcomes of a randomized clinical trial. Veterans Affairs Study of Angioplasty Compared to Medical Therapy Investigators. Circulation 1995; 92:1710–1719.
- Coylewright M, Dick S, Zmolek B, et al. PCI choice decision aid for stable coronary artery disease: a randomized trial. Circ Cardiovasc Qual Outcomes 2016; 9:767–776.
- Fontana M, Asaria P, Moraldo M, et al. Patient-accessible tool for shared decision making in cardiovascular primary prevention: balancing longevity benefits against medication disutility. Circulation 2014; 129:2539–2546.
- Weintraub WS, Boden WE, Zhang Z, et al. Cost-effectiveness of percutaneous coronary intervention in optimally treated stable coronary patients. Circ Cardiovasc Qual Outcomes 2008; 1:12–20.
- Al-Lamee R, Thompson D, Dehbi H-M, et al. Percutaneous coronary intervention in stable angina (ORBITA): a double-blind, randomised controlled trial. Lancet November 2, 2017; doi:10.1016/S0140-6736(17)32714-9.
- Fearon WF, Nishi T, Bruyne BD, et al. Clinical outcomes and cost-effectiveness of fractional flow reserve-guided percutaneous coronary intervention in patients with stable coronary artery disease: three-year follow-up of the FAME 2 Trial (Fractional Flow Reserve Versus Angiography for Multivessel Evaluation). Circulation November 2017; doi:10.1161/CIRCULATIONAHA.117.031907.
- Meier B. The first patient to undergo coronary angioplasty—23-year follow-up. N Engl J Med 2001; 344:144–145.
- Levine GN, Bates ER, Blankenship JC, et al. 2011 ACCF/AHA/SCAI Guideline for Percutaneous Coronary Intervention: executive summary: a report of the American College of Cardiology Foundation/American Heart Association Task Force on Practice Guidelines and the Society for Cardiovascular Angiography and Interventions. Circulation 2011; 124:2574–2609.
- Fox KAA, Clayton TC, Damman P, et al. Long-term outcome of a routine versus selective invasive strategy in patients with non-ST-segment elevation acute coronary syndrome a meta-analysis of individual patient data. J Am Coll Cardiol 2010; 55:2435–2445.
- Katritsis DG, Ioannidis JPA. Percutaneous coronary intervention versus conservative therapy in nonacute coronary artery disease: a meta-analysis. Circulation 2005; 111:2906–2912.
- Boden WE, O’Rourke RA, Teo KK, et al. Optimal medical therapy with or without PCI for stable coronary disease. N Engl J Med 2007; 356:1503–1516.
- Bangalore S, Gupta N, Généreux P, Guo Y, Pancholy S, Feit F. Trend in percutaneous coronary intervention volume following the COURAGE and BARI-2D trials: insight from over 8.1 million percutaneous coronary interventions. Int J Cardiol 2015; 183:6–10.
- Rothberg MB. PCI for stable angina: a missed opportunity for shared decision-making. Cleve Clin J Med 2018; 85:105–121.
- Spertus JA, Maron DJ, Cohen DJ, et al. Frequency, predictors, and consequences of crossing over to revascularization within 12 months of randomization to optimal medical therapy in the Clinical Outcomes Utilizing Revascularization and Aggressive Drug Evaluation (COURAGE) trial. Circ Cardiovasc Qual Outcomes 2013; 6:409–418.
- Weintraub WS, Spertus JA, Kolm P, et al. Effect of PCI on quality of life in patients with stable coronary disease. N Engl J Med 2008; 359:677–687.
- Acharjee S, Teo KK, Jacobs AK, et al. Optimal medical therapy with or without percutaneous coronary intervention in women with stable coronary disease: a pre-specified subset analysis of the Clinical Outcomes Utilizing Revascularization and Aggressive druG Evaluation (COURAGE) trial. Am Heart J 2016; 173:108–117.
- Foster C, Jackson AS, Pollock ML, et al. Generalized equations for predicting functional capacity from treadmill performance. Am Heart J 1984; 107:1229–1234.
- Morris CK, Myers J, Froelicher VF, Kawaguchi T, Ueshima K, Hideg A. Nomogram based on metabolic equivalents and age for assessing aerobic exercise capacity in men. J Am Coll Cardiol 1993; 22:175–182.
- Jetté M, Sidney K, Blümchen G. Metabolic equivalents (METS) in exercise testing, exercise prescription, and evaluation of functional capacity. Clin Cardiol 1990; 13:555–565.
- Erne P, Schoenenberger AW, Burckhardt D, et al. Effects of percutaneous coronary interventions in silent ischemia after myocardial infarction: the SWISSI II randomized controlled trial. JAMA 2007; 297:1985–1991.
- Strauss WE, Fortin T, Hartigan P, Folland ED, Parisi AF. A comparison of quality of life scores in patients with angina pectoris after angioplasty compared with after medical therapy. Outcomes of a randomized clinical trial. Veterans Affairs Study of Angioplasty Compared to Medical Therapy Investigators. Circulation 1995; 92:1710–1719.
- Coylewright M, Dick S, Zmolek B, et al. PCI choice decision aid for stable coronary artery disease: a randomized trial. Circ Cardiovasc Qual Outcomes 2016; 9:767–776.
- Fontana M, Asaria P, Moraldo M, et al. Patient-accessible tool for shared decision making in cardiovascular primary prevention: balancing longevity benefits against medication disutility. Circulation 2014; 129:2539–2546.
- Weintraub WS, Boden WE, Zhang Z, et al. Cost-effectiveness of percutaneous coronary intervention in optimally treated stable coronary patients. Circ Cardiovasc Qual Outcomes 2008; 1:12–20.
- Al-Lamee R, Thompson D, Dehbi H-M, et al. Percutaneous coronary intervention in stable angina (ORBITA): a double-blind, randomised controlled trial. Lancet November 2, 2017; doi:10.1016/S0140-6736(17)32714-9.
- Fearon WF, Nishi T, Bruyne BD, et al. Clinical outcomes and cost-effectiveness of fractional flow reserve-guided percutaneous coronary intervention in patients with stable coronary artery disease: three-year follow-up of the FAME 2 Trial (Fractional Flow Reserve Versus Angiography for Multivessel Evaluation). Circulation November 2017; doi:10.1161/CIRCULATIONAHA.117.031907.
Finding balance: Optimizing medication prescribing in older patients
According to a 2016 study, more than one-third of older adults in the United States take 5 or more medications.1 This is a growing problem. Not only do older patients take more drugs than younger patients, they are also at higher risk of adverse drug events, drug-drug interactions, geriatric syndromes, and lower adherence.2
Many drugs that older patients are given are potentially inappropriate, ie, their risks outweigh the expected benefits, particularly when effective and safer alternative therapies exist. Although many clinicians are aware of the risks of polypharmacy, they may not be confident in discontinuing potentially inappropriate medications. The process of deliberately tapering, stopping, or reducing doses of medications with the goal of reducing harm and improving patient outcomes is known as deprescribing.3
In this issue, Kim et al4 review several medications that are overused or often used inappropriately in older adults: statins for primary prevention of atherosclerotic cardiovascular disease, anticholinergic drugs, benzodiazepines, antipsychotics, and proton pump inhibitors. They offer guidance about the situations in which these drugs may be inappropriate as well as alternative drug and nondrug treatments. Further, they suggest that, when prescribing or deprescribing drugs in older adults, clinicians consult tools such as the Beers criteria and the STOPP/START criteria (the Screening Tool of Older Persons’ Potentially Inappropriate Prescriptions, and the Screening Tool to Alert Doctors to Right Treatment).
The issues Kim et al review are highly relevant and may increase awareness of specific potentially inappropriate medications. They also remind us that nonpharmacologic treatments are first-line for many medical conditions. In an era of a pill for every ill and a quick-fix mentality among both patients and providers, lifestyle changes and other nonpharmacologic treatments may be overlooked. Similarly, the STOPP/START criteria, which are concrete, evidence-based recommendations that can be applied to patient care, are likely underused in clinical practice.
Although necessary and valuable, simply arming clinicians with knowledge is insufficient to tackle the problems of polypharmacy and inappropriate prescribing. As the authors note in their discussion of benzodiazepines, practice guidelines exist regarding prescribing these agents, and data from randomized trials support specific interventions to deprescribe them.5 Nevertheless, clinicians report feeling inadequately prepared to discontinue benzodiazepines, particularly when patients perceive benefit from them. As such, user-friendly tools and specific strategies for weighing risks vs benefits are critical for clinicians.
PUTTING KNOWLEDGE INTO PRACTICE
How do we translate knowledge into practice with regard to deprescribing potentially inappropriate medications in older patients—or prescribing drugs only if appropriate in the first place?
An opportunity arises when patients are in the hospital. Taking a medication history on admission and matching medications with indications are key starting points. Clinical pharmacists can help screen for side effects and potential interactions and can provide deprescribing recommendations. Meticulous discharge medication reconciliation, patient education, and communication of the updated medication list to the outpatient provider are central to ensuring that patients adhere to medication adjustments after they go home.
A MATTER OF BALANCE
Another factor to consider is the patient’s physiologic age compared with his or her chronologic age. If a patient has multiple comorbidities, frailty, limited life expectancy, or poor renal function, we may consider her older than her chronologic age. In this case, a drug’s risks may outweigh its benefit, which is something to be discussed. On the other hand, a high-functioning and relatively healthy elderly patient may be a candidate for medications known to reduce the risk of death or control a chronic disease better. Incorporating a patient’s goals of care and using shared decision-making are also likely to yield an optimal medication regimen.
Smartphone apps and resources embedded in electronic health records provide additional decision support. Used when prescribing or reconciling medications, these supplemental brains offer instant feedback and information on dose adjustments, drug interactions, clinical guidelines, and even potentially inappropriate medications. While the impacts of these electronic tools on prescribing patterns and outcomes in geriatric populations remain unclear, new ones are being developed and studied.6 This may be the most promising way to translate knowledge into practice, as it is more easily integrated with existing clinician workflows.
AN OPPORTUNITY TO IMPROVE
There is significant opportunity to reduce polypharmacy and optimize medication prescribing practices for older adults. Awareness of potentially inappropriate medications and clinical situations in which the use of certain classes of medications should be minimized is the first step in addressing this problem. Using tools such as the STOPP/START criteria, reviewing medications at critical transition points, prioritizing patient function and goals, and using electronic clinical decision support should aid prescribing decisions.
Whenever possible, collaborating with other care team members such as pharmacists may increase efficiency and effectiveness of medication management. Ultimately, inclusion of more older adults in clinical trials may provide data-driven guidance for weighing risks and benefits. Finally, further study of the effects of deprescribing on clinical outcomes may be the missing piece to help clinicians and patients find balance in prescription management.
- Qato DM, Wilder J, Schumm LP, Gillet V, Alexander GC. Changes in prescription and over-the-counter medication and dietary supplement use among older adults in the United States, 2005 vs 2011. JAMA Intern Med 2016; 176:473–482.
- Saraf AA, Petersen AW, Simmons SF, et al. Medications associated with geriatric syndromes and their prevalence in older hospitalized adults discharged to skilled nursing facilities. J Hosp Med 2016; 11:694–700.
- Scott IA, Hilmer SN, Reeve E, et al. Reducing inappropriate polypharmacy: the process of deprescribing. JAMA Intern Med 2015; 175:827–834.
- Kim LD, Koncilja K, Nielsen C. Medication management in older adults. Cleve Clin J Med 2018; 85:129–135.
- Tannenbaum C, Martin P, Tamblyn R, Benedetti A, Ahmed S. Reduction of inappropriate benzodiazepine prescriptions among older adults through direct patient education: the EMPOWER cluster randomized trial. JAMA Intern Med 2014; 174:890–898.
- Alagiakrishnan K, Wilson P, Sadowski CA, et al. Physicians’ use of computerized clinical decision supports to improve medication management in the elderly—the Seniors Medication Alert and Review Technology intervention. Clin Interv Aging 2016; 11:73–81.
According to a 2016 study, more than one-third of older adults in the United States take 5 or more medications.1 This is a growing problem. Not only do older patients take more drugs than younger patients, they are also at higher risk of adverse drug events, drug-drug interactions, geriatric syndromes, and lower adherence.2
Many drugs that older patients are given are potentially inappropriate, ie, their risks outweigh the expected benefits, particularly when effective and safer alternative therapies exist. Although many clinicians are aware of the risks of polypharmacy, they may not be confident in discontinuing potentially inappropriate medications. The process of deliberately tapering, stopping, or reducing doses of medications with the goal of reducing harm and improving patient outcomes is known as deprescribing.3
In this issue, Kim et al4 review several medications that are overused or often used inappropriately in older adults: statins for primary prevention of atherosclerotic cardiovascular disease, anticholinergic drugs, benzodiazepines, antipsychotics, and proton pump inhibitors. They offer guidance about the situations in which these drugs may be inappropriate as well as alternative drug and nondrug treatments. Further, they suggest that, when prescribing or deprescribing drugs in older adults, clinicians consult tools such as the Beers criteria and the STOPP/START criteria (the Screening Tool of Older Persons’ Potentially Inappropriate Prescriptions, and the Screening Tool to Alert Doctors to Right Treatment).
The issues Kim et al review are highly relevant and may increase awareness of specific potentially inappropriate medications. They also remind us that nonpharmacologic treatments are first-line for many medical conditions. In an era of a pill for every ill and a quick-fix mentality among both patients and providers, lifestyle changes and other nonpharmacologic treatments may be overlooked. Similarly, the STOPP/START criteria, which are concrete, evidence-based recommendations that can be applied to patient care, are likely underused in clinical practice.
Although necessary and valuable, simply arming clinicians with knowledge is insufficient to tackle the problems of polypharmacy and inappropriate prescribing. As the authors note in their discussion of benzodiazepines, practice guidelines exist regarding prescribing these agents, and data from randomized trials support specific interventions to deprescribe them.5 Nevertheless, clinicians report feeling inadequately prepared to discontinue benzodiazepines, particularly when patients perceive benefit from them. As such, user-friendly tools and specific strategies for weighing risks vs benefits are critical for clinicians.
PUTTING KNOWLEDGE INTO PRACTICE
How do we translate knowledge into practice with regard to deprescribing potentially inappropriate medications in older patients—or prescribing drugs only if appropriate in the first place?
An opportunity arises when patients are in the hospital. Taking a medication history on admission and matching medications with indications are key starting points. Clinical pharmacists can help screen for side effects and potential interactions and can provide deprescribing recommendations. Meticulous discharge medication reconciliation, patient education, and communication of the updated medication list to the outpatient provider are central to ensuring that patients adhere to medication adjustments after they go home.
A MATTER OF BALANCE
Another factor to consider is the patient’s physiologic age compared with his or her chronologic age. If a patient has multiple comorbidities, frailty, limited life expectancy, or poor renal function, we may consider her older than her chronologic age. In this case, a drug’s risks may outweigh its benefit, which is something to be discussed. On the other hand, a high-functioning and relatively healthy elderly patient may be a candidate for medications known to reduce the risk of death or control a chronic disease better. Incorporating a patient’s goals of care and using shared decision-making are also likely to yield an optimal medication regimen.
Smartphone apps and resources embedded in electronic health records provide additional decision support. Used when prescribing or reconciling medications, these supplemental brains offer instant feedback and information on dose adjustments, drug interactions, clinical guidelines, and even potentially inappropriate medications. While the impacts of these electronic tools on prescribing patterns and outcomes in geriatric populations remain unclear, new ones are being developed and studied.6 This may be the most promising way to translate knowledge into practice, as it is more easily integrated with existing clinician workflows.
AN OPPORTUNITY TO IMPROVE
There is significant opportunity to reduce polypharmacy and optimize medication prescribing practices for older adults. Awareness of potentially inappropriate medications and clinical situations in which the use of certain classes of medications should be minimized is the first step in addressing this problem. Using tools such as the STOPP/START criteria, reviewing medications at critical transition points, prioritizing patient function and goals, and using electronic clinical decision support should aid prescribing decisions.
Whenever possible, collaborating with other care team members such as pharmacists may increase efficiency and effectiveness of medication management. Ultimately, inclusion of more older adults in clinical trials may provide data-driven guidance for weighing risks and benefits. Finally, further study of the effects of deprescribing on clinical outcomes may be the missing piece to help clinicians and patients find balance in prescription management.
According to a 2016 study, more than one-third of older adults in the United States take 5 or more medications.1 This is a growing problem. Not only do older patients take more drugs than younger patients, they are also at higher risk of adverse drug events, drug-drug interactions, geriatric syndromes, and lower adherence.2
Many drugs that older patients are given are potentially inappropriate, ie, their risks outweigh the expected benefits, particularly when effective and safer alternative therapies exist. Although many clinicians are aware of the risks of polypharmacy, they may not be confident in discontinuing potentially inappropriate medications. The process of deliberately tapering, stopping, or reducing doses of medications with the goal of reducing harm and improving patient outcomes is known as deprescribing.3
In this issue, Kim et al4 review several medications that are overused or often used inappropriately in older adults: statins for primary prevention of atherosclerotic cardiovascular disease, anticholinergic drugs, benzodiazepines, antipsychotics, and proton pump inhibitors. They offer guidance about the situations in which these drugs may be inappropriate as well as alternative drug and nondrug treatments. Further, they suggest that, when prescribing or deprescribing drugs in older adults, clinicians consult tools such as the Beers criteria and the STOPP/START criteria (the Screening Tool of Older Persons’ Potentially Inappropriate Prescriptions, and the Screening Tool to Alert Doctors to Right Treatment).
The issues Kim et al review are highly relevant and may increase awareness of specific potentially inappropriate medications. They also remind us that nonpharmacologic treatments are first-line for many medical conditions. In an era of a pill for every ill and a quick-fix mentality among both patients and providers, lifestyle changes and other nonpharmacologic treatments may be overlooked. Similarly, the STOPP/START criteria, which are concrete, evidence-based recommendations that can be applied to patient care, are likely underused in clinical practice.
Although necessary and valuable, simply arming clinicians with knowledge is insufficient to tackle the problems of polypharmacy and inappropriate prescribing. As the authors note in their discussion of benzodiazepines, practice guidelines exist regarding prescribing these agents, and data from randomized trials support specific interventions to deprescribe them.5 Nevertheless, clinicians report feeling inadequately prepared to discontinue benzodiazepines, particularly when patients perceive benefit from them. As such, user-friendly tools and specific strategies for weighing risks vs benefits are critical for clinicians.
PUTTING KNOWLEDGE INTO PRACTICE
How do we translate knowledge into practice with regard to deprescribing potentially inappropriate medications in older patients—or prescribing drugs only if appropriate in the first place?
An opportunity arises when patients are in the hospital. Taking a medication history on admission and matching medications with indications are key starting points. Clinical pharmacists can help screen for side effects and potential interactions and can provide deprescribing recommendations. Meticulous discharge medication reconciliation, patient education, and communication of the updated medication list to the outpatient provider are central to ensuring that patients adhere to medication adjustments after they go home.
A MATTER OF BALANCE
Another factor to consider is the patient’s physiologic age compared with his or her chronologic age. If a patient has multiple comorbidities, frailty, limited life expectancy, or poor renal function, we may consider her older than her chronologic age. In this case, a drug’s risks may outweigh its benefit, which is something to be discussed. On the other hand, a high-functioning and relatively healthy elderly patient may be a candidate for medications known to reduce the risk of death or control a chronic disease better. Incorporating a patient’s goals of care and using shared decision-making are also likely to yield an optimal medication regimen.
Smartphone apps and resources embedded in electronic health records provide additional decision support. Used when prescribing or reconciling medications, these supplemental brains offer instant feedback and information on dose adjustments, drug interactions, clinical guidelines, and even potentially inappropriate medications. While the impacts of these electronic tools on prescribing patterns and outcomes in geriatric populations remain unclear, new ones are being developed and studied.6 This may be the most promising way to translate knowledge into practice, as it is more easily integrated with existing clinician workflows.
AN OPPORTUNITY TO IMPROVE
There is significant opportunity to reduce polypharmacy and optimize medication prescribing practices for older adults. Awareness of potentially inappropriate medications and clinical situations in which the use of certain classes of medications should be minimized is the first step in addressing this problem. Using tools such as the STOPP/START criteria, reviewing medications at critical transition points, prioritizing patient function and goals, and using electronic clinical decision support should aid prescribing decisions.
Whenever possible, collaborating with other care team members such as pharmacists may increase efficiency and effectiveness of medication management. Ultimately, inclusion of more older adults in clinical trials may provide data-driven guidance for weighing risks and benefits. Finally, further study of the effects of deprescribing on clinical outcomes may be the missing piece to help clinicians and patients find balance in prescription management.
- Qato DM, Wilder J, Schumm LP, Gillet V, Alexander GC. Changes in prescription and over-the-counter medication and dietary supplement use among older adults in the United States, 2005 vs 2011. JAMA Intern Med 2016; 176:473–482.
- Saraf AA, Petersen AW, Simmons SF, et al. Medications associated with geriatric syndromes and their prevalence in older hospitalized adults discharged to skilled nursing facilities. J Hosp Med 2016; 11:694–700.
- Scott IA, Hilmer SN, Reeve E, et al. Reducing inappropriate polypharmacy: the process of deprescribing. JAMA Intern Med 2015; 175:827–834.
- Kim LD, Koncilja K, Nielsen C. Medication management in older adults. Cleve Clin J Med 2018; 85:129–135.
- Tannenbaum C, Martin P, Tamblyn R, Benedetti A, Ahmed S. Reduction of inappropriate benzodiazepine prescriptions among older adults through direct patient education: the EMPOWER cluster randomized trial. JAMA Intern Med 2014; 174:890–898.
- Alagiakrishnan K, Wilson P, Sadowski CA, et al. Physicians’ use of computerized clinical decision supports to improve medication management in the elderly—the Seniors Medication Alert and Review Technology intervention. Clin Interv Aging 2016; 11:73–81.
- Qato DM, Wilder J, Schumm LP, Gillet V, Alexander GC. Changes in prescription and over-the-counter medication and dietary supplement use among older adults in the United States, 2005 vs 2011. JAMA Intern Med 2016; 176:473–482.
- Saraf AA, Petersen AW, Simmons SF, et al. Medications associated with geriatric syndromes and their prevalence in older hospitalized adults discharged to skilled nursing facilities. J Hosp Med 2016; 11:694–700.
- Scott IA, Hilmer SN, Reeve E, et al. Reducing inappropriate polypharmacy: the process of deprescribing. JAMA Intern Med 2015; 175:827–834.
- Kim LD, Koncilja K, Nielsen C. Medication management in older adults. Cleve Clin J Med 2018; 85:129–135.
- Tannenbaum C, Martin P, Tamblyn R, Benedetti A, Ahmed S. Reduction of inappropriate benzodiazepine prescriptions among older adults through direct patient education: the EMPOWER cluster randomized trial. JAMA Intern Med 2014; 174:890–898.
- Alagiakrishnan K, Wilson P, Sadowski CA, et al. Physicians’ use of computerized clinical decision supports to improve medication management in the elderly—the Seniors Medication Alert and Review Technology intervention. Clin Interv Aging 2016; 11:73–81.
Preparing from the Outside Looking In for Safely Transitioning Pediatric Inpatients to Home
The transition of children from hospital to home introduces a unique set of challenges to patients and families who may not be well-versed in the healthcare system. In addition to juggling the stress and worry of a sick child, which can inhibit the ability to understand complicated discharge instructions prior to leaving the hospital,1 caregivers need to navigate the medical system to ensure continued recovery. The responsibility to fill and administer medications, arrange follow up appointments, and determine when to seek care if the child’s condition changes are burdens we as healthcare providers expect caregivers to manage but may underestimate how frequently they are reliably completed.2-4
In this issue of the Journal of Hospital Medicine, the article by Rehm et al.5 adds to the growing body of evidence highlighting challenges that caregivers of children face upon discharge from the hospital. The multicenter, retrospective study of postdischarge encounters for over 12,000 patients discharged from 4 children’s hospitals aimed to evaluate the following: (1) various methods for hospital-initiated postdischarge contact of families, (2) the type and frequency of postdischarge issues, and (3) specific characteristics of pediatric patients most commonly affected by postdischarge issues.
Using standardized questions administered through telephone, text, or e-mail contact, postdischarge issues were identified in 25% of discharges across all hospitals. Notably, there was considerable variation of rates of postdischarge issues among hospitals (from 16% to 62.8%). The hospital with the highest rate of postdischarge issues identified had attending hospitalists calling families after discharge. Thus, postdischarge issues may be most easily identified by providers who are familiar with both the patient and the expected postdischarge care.
Often, postdischarge issues represented events that could be mitigated with intentional planning to better anticipate and address patient and family needs prior to discharge. The vast majority of postdischarge issues identified across all hospitals were related to appointments, accounting for 76.3% of postdischarge issues, which may be attributed to a variety of causes, from inadequate or unclear provider recommendations to difficulty scheduling the appointments. The most common medication postdischarge issue was difficulty filling prescriptions, accounting for 84.8% of the medication issues. “Other” postdischarge issues (12.7%) as reported by caregivers included challenges with understanding discharge instructions and concerns about changes in their child’s clinical status. Forty percent of included patients had a chronic care condition. Older children, patients with more medication classes, shorter length of stay, and neuromuscular chronic care conditions had higher odds of postdischarge issues. Although a high proportion of postdischarge issues suggests a systemic problem addressing the needs of patients and families after hospital discharge, these data likely underestimate the magnitude of the problem; as such, the need for improvement may be higher.
Postdischarge challenges faced by families are not unique to pediatrics. Pediatric and adult medical patients face similar rates of challenges after
Given the prevalence of postdischarge issues after both pediatric and adult hospitalizations, how should hospitalists proceed? Physicians and health systems should explore approaches to better prepare caregivers, perhaps using models akin to the Seamless Transitions and (Re)admissions Network model of enhanced communication, care coordination, and family engagement.10 Pediatric hospitalists can prepare children for discharge long before departure by delivering medications to patients prior to discharge,11,12 providing discharge instructions that are clear and readable,13,14 as well as utilizing admission-discharge teaching nurses,15 inpatient care managers,16,17 and pediatric nurse practitioners18 to aid transition.
While a variety of interventions show promise in securing a successful transition to home from the hospitalist vantage point, a partnership with primary care physicians (PCPs) in our communities is paramount. Though the evidence linking gaps in primary care after discharge and readmission rates remain elusive, effective partnerships with PCPs are important for ensuring discharge plans are carried out, which may ultimately lead to decreased rates of unanticipated adverse outcomes. Several adult studies note that no single intervention is likely to prevent issues after discharge, but interventions should include high-quality communication with and involvement of community partners.9,19,20 In practice, providing a high-quality, reliable handoff can be difficult given competing priorities of busy outpatient clinic schedules and inpatient bed capacity concerns, necessitating efficient discharge practices. Some of these challenges are amenable to quality improvement efforts to improve discharge communication.21 Innovative ideas include collaborating with PCPs earlier in the admission to design the care plan up front, including PCPs in weekly team meetings for patients with chronic care conditions,16,17 and using telehealth to communicate with PCPs.
Ensuring a safe transition to home is our responsibility as hospitalists, but the solutions to doing so reliably require multi-fold interventions that build teams within hospitals, innovative outreach to those patients recently discharged to ensure their well-being and mitigate postdischarge issues and broad community programs—including greater access to primary care—to meet our urgent imperative.
Disclosure
The authors declare no conflicts of interest. Dr. Auger’s research is funded by the Agency for Healthcare Research and Quality (1K08HS024735).
1. Solan LG, Beck AF, Brunswick SA, et al. The Family Perspective on Hospital to Home Transitions: A Qualitative Study. Pediatrics. 2015;136(6):e1539-1549. PubMed
2. Misky GJ, Wald HL, Coleman EA. Post-hospitalization transitions: Examining the effects of timing of primary care provider follow-up. J Hosp Med. 2010;5(7):392-397. PubMed
3. Yin HS, Johnson M, Mendelsohn AL, Abrams MA, Sanders LM, Dreyer BP. The health literacy of parents in the United States: a nationally representative study. Pediatrics. 2009;124 Suppl 3:S289-298. PubMed
4. Glick AF, Farkas JS, Nicholson J, et al. Parental Management of Discharge Instructions: A Systematic Review. Pediatrics. 2017. [Epub ahead of print]. PubMed
5. Rehm KP, Brittan MS, Stephens JR, et al. Issues Identified by Post-Discharge Contact after Pediatric Hospitalization: A Multi-site Study (published online ahead of print February 2, 2018) J Hosp Med. doi: 10.12788/jhm.2934
6. Forster AJ, Murff HJ, Peterson JF, Gandhi TK, Bates DW. The incidence and severity of adverse events affecting patients after discharge from the hospital. Ann Intern Med. 2003;138(3):161-167. PubMed
7. Hansen LO, Greenwald JL, Budnitz T, et al. Project BOOST: effectiveness of a multihospital effort to reduce rehospitalization. J Hosp Med. 2013;8(8):421-427. PubMed
8. Auerbach AD, Kripalani S, Vasilevskis EE, et al. Preventability and Causes of Readmissions in a National Cohort of General Medicine Patients. JAMA Intern Med. 2016;176(4):484-493. PubMed
9. Kripalani S, LeFevre F, Phillips CO, Williams MV, Basaviah P, Baker DW. Deficits in communication and information transfer between hospital-based and primary care physicians: implications for patient safety and continuity of care. JAMA. 2007;297(8):831-841. PubMed
10. Auger KA, Simon TD, Cooperberg D, et al. Summary of STARNet: Seamless Transitions and (Re)admissions Network. Pediatrics. 2015;135(1):164-175. PubMed
11. Hatoun J, Bair-Merritt M, Cabral H, Moses J. Increasing Medication Possession at Discharge for Patients With Asthma: The Meds-in-Hand Project. Pediatrics. 2016;137(3):e20150461. PubMed
12. White CM, Statile AM, White DL, et al. Using quality improvement to optimise paediatric discharge efficiency. BMJ Qual Saf. 2014;23(5):428-436. PubMed
13. Unaka N, Statile A, Jerardi K, et al. Improving the Readability of Pediatric Hospital Medicine Discharge Instructions. J Hosp Med. 2017;12(7):551-557. PubMed
14. Wu S, Tyler A, Logsdon T, et al. A Quality Improvement Collaborative to Improve the Discharge Process for Hospitalized Children. Pediatrics. 2016;138(2). PubMed
15. Blankenship JS, Winslow SA. Admission-discharge-teaching nurses: bridging the gap in today’s workforce. J Nurs Adm. 2003;33(1):11-13. PubMed
16. White CM, Thomson JE, Statile AM, et al. Development of a New Care Model for Hospitalized Children With Medical Complexity. Hosp Pediatr. 2017;7(7):410-414. PubMed
17. Statile AM, Schondelmeyer AC, Thomson JE, et al. Improving Discharge Efficiency in Medically Complex Pediatric Patients. Pediatrics. 2016;138(2). PubMed
18. Dunn K, Rogers J. Discharge Facilitation: An Innovative PNP Role. J Pediatr Health Care. 2016;30(5):499-505. PubMed
19. Kripalani S, Jackson AT, Schnipper JL, Coleman EA. Promoting effective transitions of care at hospital discharge: a review of key issues for hospitalists. J Hosp Med. 2007;2(5):314-323. PubMed
20. Scott AM, Li J, Oyewole-Eletu S, et al. Understanding Facilitators and Barriers to Care Transitions: Insights from Project ACHIEVE Site Visits. Jt Comm J Qual Patient Saf. 2017;43(9):433-447. PubMed
21. Shen MW, Hershey D, Bergert L, Mallory L, Fisher ES, Cooperberg D. Pediatric hospitalists collaborate to improve timeliness of discharge communication. Hosp Pediatr. 2013;3(3):258-265. PubMed
The transition of children from hospital to home introduces a unique set of challenges to patients and families who may not be well-versed in the healthcare system. In addition to juggling the stress and worry of a sick child, which can inhibit the ability to understand complicated discharge instructions prior to leaving the hospital,1 caregivers need to navigate the medical system to ensure continued recovery. The responsibility to fill and administer medications, arrange follow up appointments, and determine when to seek care if the child’s condition changes are burdens we as healthcare providers expect caregivers to manage but may underestimate how frequently they are reliably completed.2-4
In this issue of the Journal of Hospital Medicine, the article by Rehm et al.5 adds to the growing body of evidence highlighting challenges that caregivers of children face upon discharge from the hospital. The multicenter, retrospective study of postdischarge encounters for over 12,000 patients discharged from 4 children’s hospitals aimed to evaluate the following: (1) various methods for hospital-initiated postdischarge contact of families, (2) the type and frequency of postdischarge issues, and (3) specific characteristics of pediatric patients most commonly affected by postdischarge issues.
Using standardized questions administered through telephone, text, or e-mail contact, postdischarge issues were identified in 25% of discharges across all hospitals. Notably, there was considerable variation of rates of postdischarge issues among hospitals (from 16% to 62.8%). The hospital with the highest rate of postdischarge issues identified had attending hospitalists calling families after discharge. Thus, postdischarge issues may be most easily identified by providers who are familiar with both the patient and the expected postdischarge care.
Often, postdischarge issues represented events that could be mitigated with intentional planning to better anticipate and address patient and family needs prior to discharge. The vast majority of postdischarge issues identified across all hospitals were related to appointments, accounting for 76.3% of postdischarge issues, which may be attributed to a variety of causes, from inadequate or unclear provider recommendations to difficulty scheduling the appointments. The most common medication postdischarge issue was difficulty filling prescriptions, accounting for 84.8% of the medication issues. “Other” postdischarge issues (12.7%) as reported by caregivers included challenges with understanding discharge instructions and concerns about changes in their child’s clinical status. Forty percent of included patients had a chronic care condition. Older children, patients with more medication classes, shorter length of stay, and neuromuscular chronic care conditions had higher odds of postdischarge issues. Although a high proportion of postdischarge issues suggests a systemic problem addressing the needs of patients and families after hospital discharge, these data likely underestimate the magnitude of the problem; as such, the need for improvement may be higher.
Postdischarge challenges faced by families are not unique to pediatrics. Pediatric and adult medical patients face similar rates of challenges after
Given the prevalence of postdischarge issues after both pediatric and adult hospitalizations, how should hospitalists proceed? Physicians and health systems should explore approaches to better prepare caregivers, perhaps using models akin to the Seamless Transitions and (Re)admissions Network model of enhanced communication, care coordination, and family engagement.10 Pediatric hospitalists can prepare children for discharge long before departure by delivering medications to patients prior to discharge,11,12 providing discharge instructions that are clear and readable,13,14 as well as utilizing admission-discharge teaching nurses,15 inpatient care managers,16,17 and pediatric nurse practitioners18 to aid transition.
While a variety of interventions show promise in securing a successful transition to home from the hospitalist vantage point, a partnership with primary care physicians (PCPs) in our communities is paramount. Though the evidence linking gaps in primary care after discharge and readmission rates remain elusive, effective partnerships with PCPs are important for ensuring discharge plans are carried out, which may ultimately lead to decreased rates of unanticipated adverse outcomes. Several adult studies note that no single intervention is likely to prevent issues after discharge, but interventions should include high-quality communication with and involvement of community partners.9,19,20 In practice, providing a high-quality, reliable handoff can be difficult given competing priorities of busy outpatient clinic schedules and inpatient bed capacity concerns, necessitating efficient discharge practices. Some of these challenges are amenable to quality improvement efforts to improve discharge communication.21 Innovative ideas include collaborating with PCPs earlier in the admission to design the care plan up front, including PCPs in weekly team meetings for patients with chronic care conditions,16,17 and using telehealth to communicate with PCPs.
Ensuring a safe transition to home is our responsibility as hospitalists, but the solutions to doing so reliably require multi-fold interventions that build teams within hospitals, innovative outreach to those patients recently discharged to ensure their well-being and mitigate postdischarge issues and broad community programs—including greater access to primary care—to meet our urgent imperative.
Disclosure
The authors declare no conflicts of interest. Dr. Auger’s research is funded by the Agency for Healthcare Research and Quality (1K08HS024735).
The transition of children from hospital to home introduces a unique set of challenges to patients and families who may not be well-versed in the healthcare system. In addition to juggling the stress and worry of a sick child, which can inhibit the ability to understand complicated discharge instructions prior to leaving the hospital,1 caregivers need to navigate the medical system to ensure continued recovery. The responsibility to fill and administer medications, arrange follow up appointments, and determine when to seek care if the child’s condition changes are burdens we as healthcare providers expect caregivers to manage but may underestimate how frequently they are reliably completed.2-4
In this issue of the Journal of Hospital Medicine, the article by Rehm et al.5 adds to the growing body of evidence highlighting challenges that caregivers of children face upon discharge from the hospital. The multicenter, retrospective study of postdischarge encounters for over 12,000 patients discharged from 4 children’s hospitals aimed to evaluate the following: (1) various methods for hospital-initiated postdischarge contact of families, (2) the type and frequency of postdischarge issues, and (3) specific characteristics of pediatric patients most commonly affected by postdischarge issues.
Using standardized questions administered through telephone, text, or e-mail contact, postdischarge issues were identified in 25% of discharges across all hospitals. Notably, there was considerable variation of rates of postdischarge issues among hospitals (from 16% to 62.8%). The hospital with the highest rate of postdischarge issues identified had attending hospitalists calling families after discharge. Thus, postdischarge issues may be most easily identified by providers who are familiar with both the patient and the expected postdischarge care.
Often, postdischarge issues represented events that could be mitigated with intentional planning to better anticipate and address patient and family needs prior to discharge. The vast majority of postdischarge issues identified across all hospitals were related to appointments, accounting for 76.3% of postdischarge issues, which may be attributed to a variety of causes, from inadequate or unclear provider recommendations to difficulty scheduling the appointments. The most common medication postdischarge issue was difficulty filling prescriptions, accounting for 84.8% of the medication issues. “Other” postdischarge issues (12.7%) as reported by caregivers included challenges with understanding discharge instructions and concerns about changes in their child’s clinical status. Forty percent of included patients had a chronic care condition. Older children, patients with more medication classes, shorter length of stay, and neuromuscular chronic care conditions had higher odds of postdischarge issues. Although a high proportion of postdischarge issues suggests a systemic problem addressing the needs of patients and families after hospital discharge, these data likely underestimate the magnitude of the problem; as such, the need for improvement may be higher.
Postdischarge challenges faced by families are not unique to pediatrics. Pediatric and adult medical patients face similar rates of challenges after
Given the prevalence of postdischarge issues after both pediatric and adult hospitalizations, how should hospitalists proceed? Physicians and health systems should explore approaches to better prepare caregivers, perhaps using models akin to the Seamless Transitions and (Re)admissions Network model of enhanced communication, care coordination, and family engagement.10 Pediatric hospitalists can prepare children for discharge long before departure by delivering medications to patients prior to discharge,11,12 providing discharge instructions that are clear and readable,13,14 as well as utilizing admission-discharge teaching nurses,15 inpatient care managers,16,17 and pediatric nurse practitioners18 to aid transition.
While a variety of interventions show promise in securing a successful transition to home from the hospitalist vantage point, a partnership with primary care physicians (PCPs) in our communities is paramount. Though the evidence linking gaps in primary care after discharge and readmission rates remain elusive, effective partnerships with PCPs are important for ensuring discharge plans are carried out, which may ultimately lead to decreased rates of unanticipated adverse outcomes. Several adult studies note that no single intervention is likely to prevent issues after discharge, but interventions should include high-quality communication with and involvement of community partners.9,19,20 In practice, providing a high-quality, reliable handoff can be difficult given competing priorities of busy outpatient clinic schedules and inpatient bed capacity concerns, necessitating efficient discharge practices. Some of these challenges are amenable to quality improvement efforts to improve discharge communication.21 Innovative ideas include collaborating with PCPs earlier in the admission to design the care plan up front, including PCPs in weekly team meetings for patients with chronic care conditions,16,17 and using telehealth to communicate with PCPs.
Ensuring a safe transition to home is our responsibility as hospitalists, but the solutions to doing so reliably require multi-fold interventions that build teams within hospitals, innovative outreach to those patients recently discharged to ensure their well-being and mitigate postdischarge issues and broad community programs—including greater access to primary care—to meet our urgent imperative.
Disclosure
The authors declare no conflicts of interest. Dr. Auger’s research is funded by the Agency for Healthcare Research and Quality (1K08HS024735).
1. Solan LG, Beck AF, Brunswick SA, et al. The Family Perspective on Hospital to Home Transitions: A Qualitative Study. Pediatrics. 2015;136(6):e1539-1549. PubMed
2. Misky GJ, Wald HL, Coleman EA. Post-hospitalization transitions: Examining the effects of timing of primary care provider follow-up. J Hosp Med. 2010;5(7):392-397. PubMed
3. Yin HS, Johnson M, Mendelsohn AL, Abrams MA, Sanders LM, Dreyer BP. The health literacy of parents in the United States: a nationally representative study. Pediatrics. 2009;124 Suppl 3:S289-298. PubMed
4. Glick AF, Farkas JS, Nicholson J, et al. Parental Management of Discharge Instructions: A Systematic Review. Pediatrics. 2017. [Epub ahead of print]. PubMed
5. Rehm KP, Brittan MS, Stephens JR, et al. Issues Identified by Post-Discharge Contact after Pediatric Hospitalization: A Multi-site Study (published online ahead of print February 2, 2018) J Hosp Med. doi: 10.12788/jhm.2934
6. Forster AJ, Murff HJ, Peterson JF, Gandhi TK, Bates DW. The incidence and severity of adverse events affecting patients after discharge from the hospital. Ann Intern Med. 2003;138(3):161-167. PubMed
7. Hansen LO, Greenwald JL, Budnitz T, et al. Project BOOST: effectiveness of a multihospital effort to reduce rehospitalization. J Hosp Med. 2013;8(8):421-427. PubMed
8. Auerbach AD, Kripalani S, Vasilevskis EE, et al. Preventability and Causes of Readmissions in a National Cohort of General Medicine Patients. JAMA Intern Med. 2016;176(4):484-493. PubMed
9. Kripalani S, LeFevre F, Phillips CO, Williams MV, Basaviah P, Baker DW. Deficits in communication and information transfer between hospital-based and primary care physicians: implications for patient safety and continuity of care. JAMA. 2007;297(8):831-841. PubMed
10. Auger KA, Simon TD, Cooperberg D, et al. Summary of STARNet: Seamless Transitions and (Re)admissions Network. Pediatrics. 2015;135(1):164-175. PubMed
11. Hatoun J, Bair-Merritt M, Cabral H, Moses J. Increasing Medication Possession at Discharge for Patients With Asthma: The Meds-in-Hand Project. Pediatrics. 2016;137(3):e20150461. PubMed
12. White CM, Statile AM, White DL, et al. Using quality improvement to optimise paediatric discharge efficiency. BMJ Qual Saf. 2014;23(5):428-436. PubMed
13. Unaka N, Statile A, Jerardi K, et al. Improving the Readability of Pediatric Hospital Medicine Discharge Instructions. J Hosp Med. 2017;12(7):551-557. PubMed
14. Wu S, Tyler A, Logsdon T, et al. A Quality Improvement Collaborative to Improve the Discharge Process for Hospitalized Children. Pediatrics. 2016;138(2). PubMed
15. Blankenship JS, Winslow SA. Admission-discharge-teaching nurses: bridging the gap in today’s workforce. J Nurs Adm. 2003;33(1):11-13. PubMed
16. White CM, Thomson JE, Statile AM, et al. Development of a New Care Model for Hospitalized Children With Medical Complexity. Hosp Pediatr. 2017;7(7):410-414. PubMed
17. Statile AM, Schondelmeyer AC, Thomson JE, et al. Improving Discharge Efficiency in Medically Complex Pediatric Patients. Pediatrics. 2016;138(2). PubMed
18. Dunn K, Rogers J. Discharge Facilitation: An Innovative PNP Role. J Pediatr Health Care. 2016;30(5):499-505. PubMed
19. Kripalani S, Jackson AT, Schnipper JL, Coleman EA. Promoting effective transitions of care at hospital discharge: a review of key issues for hospitalists. J Hosp Med. 2007;2(5):314-323. PubMed
20. Scott AM, Li J, Oyewole-Eletu S, et al. Understanding Facilitators and Barriers to Care Transitions: Insights from Project ACHIEVE Site Visits. Jt Comm J Qual Patient Saf. 2017;43(9):433-447. PubMed
21. Shen MW, Hershey D, Bergert L, Mallory L, Fisher ES, Cooperberg D. Pediatric hospitalists collaborate to improve timeliness of discharge communication. Hosp Pediatr. 2013;3(3):258-265. PubMed
1. Solan LG, Beck AF, Brunswick SA, et al. The Family Perspective on Hospital to Home Transitions: A Qualitative Study. Pediatrics. 2015;136(6):e1539-1549. PubMed
2. Misky GJ, Wald HL, Coleman EA. Post-hospitalization transitions: Examining the effects of timing of primary care provider follow-up. J Hosp Med. 2010;5(7):392-397. PubMed
3. Yin HS, Johnson M, Mendelsohn AL, Abrams MA, Sanders LM, Dreyer BP. The health literacy of parents in the United States: a nationally representative study. Pediatrics. 2009;124 Suppl 3:S289-298. PubMed
4. Glick AF, Farkas JS, Nicholson J, et al. Parental Management of Discharge Instructions: A Systematic Review. Pediatrics. 2017. [Epub ahead of print]. PubMed
5. Rehm KP, Brittan MS, Stephens JR, et al. Issues Identified by Post-Discharge Contact after Pediatric Hospitalization: A Multi-site Study (published online ahead of print February 2, 2018) J Hosp Med. doi: 10.12788/jhm.2934
6. Forster AJ, Murff HJ, Peterson JF, Gandhi TK, Bates DW. The incidence and severity of adverse events affecting patients after discharge from the hospital. Ann Intern Med. 2003;138(3):161-167. PubMed
7. Hansen LO, Greenwald JL, Budnitz T, et al. Project BOOST: effectiveness of a multihospital effort to reduce rehospitalization. J Hosp Med. 2013;8(8):421-427. PubMed
8. Auerbach AD, Kripalani S, Vasilevskis EE, et al. Preventability and Causes of Readmissions in a National Cohort of General Medicine Patients. JAMA Intern Med. 2016;176(4):484-493. PubMed
9. Kripalani S, LeFevre F, Phillips CO, Williams MV, Basaviah P, Baker DW. Deficits in communication and information transfer between hospital-based and primary care physicians: implications for patient safety and continuity of care. JAMA. 2007;297(8):831-841. PubMed
10. Auger KA, Simon TD, Cooperberg D, et al. Summary of STARNet: Seamless Transitions and (Re)admissions Network. Pediatrics. 2015;135(1):164-175. PubMed
11. Hatoun J, Bair-Merritt M, Cabral H, Moses J. Increasing Medication Possession at Discharge for Patients With Asthma: The Meds-in-Hand Project. Pediatrics. 2016;137(3):e20150461. PubMed
12. White CM, Statile AM, White DL, et al. Using quality improvement to optimise paediatric discharge efficiency. BMJ Qual Saf. 2014;23(5):428-436. PubMed
13. Unaka N, Statile A, Jerardi K, et al. Improving the Readability of Pediatric Hospital Medicine Discharge Instructions. J Hosp Med. 2017;12(7):551-557. PubMed
14. Wu S, Tyler A, Logsdon T, et al. A Quality Improvement Collaborative to Improve the Discharge Process for Hospitalized Children. Pediatrics. 2016;138(2). PubMed
15. Blankenship JS, Winslow SA. Admission-discharge-teaching nurses: bridging the gap in today’s workforce. J Nurs Adm. 2003;33(1):11-13. PubMed
16. White CM, Thomson JE, Statile AM, et al. Development of a New Care Model for Hospitalized Children With Medical Complexity. Hosp Pediatr. 2017;7(7):410-414. PubMed
17. Statile AM, Schondelmeyer AC, Thomson JE, et al. Improving Discharge Efficiency in Medically Complex Pediatric Patients. Pediatrics. 2016;138(2). PubMed
18. Dunn K, Rogers J. Discharge Facilitation: An Innovative PNP Role. J Pediatr Health Care. 2016;30(5):499-505. PubMed
19. Kripalani S, Jackson AT, Schnipper JL, Coleman EA. Promoting effective transitions of care at hospital discharge: a review of key issues for hospitalists. J Hosp Med. 2007;2(5):314-323. PubMed
20. Scott AM, Li J, Oyewole-Eletu S, et al. Understanding Facilitators and Barriers to Care Transitions: Insights from Project ACHIEVE Site Visits. Jt Comm J Qual Patient Saf. 2017;43(9):433-447. PubMed
21. Shen MW, Hershey D, Bergert L, Mallory L, Fisher ES, Cooperberg D. Pediatric hospitalists collaborate to improve timeliness of discharge communication. Hosp Pediatr. 2013;3(3):258-265. PubMed
© 2018 Society of Hospital Medicine